3D printing in neurosurgery
3D printing in neurosurgery: A systematic review
1. Abla AA, Lawton MT. Three-dimensional hollow intracranial aneurysm models and their potential role for teaching, simulation, and training. World Neurosurg. 2015;83:35–6. [PubMed] [Google Scholar]
2. Anderson JR, Thompson WL, Alkattan AK, Diaz O, Klucznik R, Zhang YJ, et al. Three-dimensional printing of anatomically accurate, patient specific intracranial aneurysm models. J Neurointerv Surg. 2016;8:517–20. [PubMed] [Google Scholar]
3. Bova FJ, Rajon DA, Friedman WA, Murad GJ, Hoh DJ, Jacob RP, et al. Mixed-reality simulation for neurosurgical procedures. Neurosurgery. 2013;73:138–45. [PubMed] [Google Scholar]
4. Gatto M, Memoli G, Shaw A, Sadhoo N, Gelat P, Harris RA. Three-Dimensional Printing (3DP) of neonatal head phantom for ultrasound: Thermocouple embedding and simulation of bone. Med Eng Phys. 2012;34:929–37. [PubMed] [Google Scholar]
5. Hirata M, Morris S, Sugata H, Matsushita K, Yanagisawa T, Kishima H, et al. Patient-specific contour-fitting sheet electrodes for electrocorticographic brain machine interfaces. Conf Proc IEEE Eng Med Biol Soc 2014. 2014:5204–7. [PubMed] [Google Scholar]
6. Inoue D, Yoshimoto K, Uemura M, Yoshida M, Ohuchida K, Kenmotsu H, et al. Three-dimensional high-definition neuroendoscopic surgery: A controlled comparative laboratory study with two-dimensional endoscopy and clinical application. J Neurol Surg A Cent Eur Neurosurg. 2013;74:357–65. [PubMed] [Google Scholar]
7. Ionita CN, Mokin M, Varble N, Bednarek DR, Xiang J, Snyder KV, et al. Challenges and limitations of patient-specific vascular phantom fabrication using 3D Polyjet printing. Proc SPIE Int Soc Opt Eng. 2014;9038:90380M. [PMC free article] [PubMed] [Google Scholar]
8. Ju SG, Kim MK, Hong CS, Kim JS, Han Y, Choi DH, et al. New technique for developing a proton range compensator with use of a 3-dimensional printer. Int J Radiat Oncol Biol Phys. 2014;88:453–8. [PubMed] [Google Scholar]
9. Khan IS, Kelly PD, Singer RJ. Prototyping of cerebral vasculature physical models. Surg Neurol Int. 2014;5:11. [PMC free article] [PubMed] [Google Scholar]
10. Klein GT, Lu Y, Wang MY. 3D Printing and Neurosurgery—Ready for Prime Time? World Neurosurg. 2013;80:233–5. [PubMed] [Google Scholar]
11. Kondo K1, Nemoto M, Masuda H, Okonogi S, Nomoto J, Harada N, et al. Anatomical Reproducibility of a Head Model Molded by a Three-dimensional Printer. Neurol Med Chir. 2015;55:592–8. [PMC free article] [PubMed] [Google Scholar]
12. Li Z, Li Z, Xu R, Li M, Li J, Liu Y, et al. Three-dimensional printing models improve understanding of spinal fracture—A randomized controlled study in China. Sci Rep. 2015;5:11570. [PMC free article] [PubMed] [Google Scholar]
13. Liew Y, Beveridge E, Demetriades AK, Hughes MA. 3D printing of patient-specific anatomy: A tool to improve patient consent and enhance imaging interpretation by trainees. Br J Neurosurg. 2015;29:712–4. [PubMed] [Google Scholar]
14. Mashiko T, Otani K, Kawano R, Konno T, Kaneko N, Ito Y, et al. Development of three-dimensional hollow elastic model for cerebral aneurysm clipping simulation enabling rapid and low cost prototyping. World Neurosurg. 2015;83:351–61. [PubMed] [Google Scholar]
15. Mashiko T, Konno T, Kaneko N, Watanabe E. Training in Brain Retraction Using a Self-Made Three-Dimensional Model. World Neurosurg. 2015;84:585–90. [PubMed] [Google Scholar]
16. Menikou G, Dadakova T, Pavlina M, Bock M, Damianou C. MRI compatible head phantom for ultrasound surgery. Ultrasonics. 2015;57:144–52. [PubMed] [Google Scholar]
17. Morris S, Hirata M, Sugata H, Goto T, Matsushita K, Yanagisawa T, et al. Patient Specific Cortical Electrodes for Sulcal and Gyral Implantation. IEEE Trans Biomed Eng. 2014;62:1034–41. [PubMed] [Google Scholar]
18. Naftulin JS, Kimchi EY, Cash SS. Streamlined, Inexpensive 3D Printing of the Brain and Skull. PLoS One. 2015;10:e0136198. [PMC free article] [PubMed] [Google Scholar]
19. Namba K, Higaki A, Kaneko N, Mashiko T, Nemoto S, Watanabe E. Microcatheter Shaping for Intracranial Aneurysm Coiling Using the 3-Dimensional Printing Rapid Prototyping Technology: Preliminary Result in the First 10 Consecutive Cases. World Neurosurg. 2015;84:178–86. [PubMed] [Google Scholar]
20. Oishi M, Fukuda M, Yajima N, Yoshida K, Takahashi M, Hiraishi T, et al. Interactive presurgical simulation applying advanced 3D imaging and modeling techniques for skull base and deep tumors. J Neurosurg. 2013;119:94–105. [PubMed] [Google Scholar]
21. Ryan JR, Chen T, Nakaji P, Frakes DH, Gonzalez LF. Ventriculostomy Simulation Using Patient-specific ventricular anatomy, 3D Printing, and Hydrogel Casting. World Neurosurg. 2015;84:1333–9. [PubMed] [Google Scholar]
22. Spottiswoode BS, Van Den Heever DJ, Chang Y, Engelhardt S, Du Plessis S, Nicolls F, et al. Preoperative three-dimensional model creation of magnetic resonance brain images as a tool to assist neurosurgical planning. Stereotact Funct Neurosurg. 2013;91:162–9. [PubMed] [Google Scholar]
23. Sugawara T, Higashiyama N, Kaneyama S, Takabatake M, Watanabe N, Uchida F, et al. Multistep pedicle screw insertion procedure with patient-specific lamina fit-and-lock templates for the thoracic spine. J Neurosurg Spine. 2013;19:185–90. [PubMed] [Google Scholar]
24. Tai BL, Rooney D, Stephenson F, Liao P, Sagher O, Shih AJ, et al. Development of a 3D-printed external ventricular drain placement simulator: Technical note. J Neurosurg. 2015;123:1–7. [PubMed] [Google Scholar]
25. Tan ET, Ling JM, Dinesh SK. The feasibility of producing patient-specific acrylic cranioplasty implants with a low-cost 3D printer. J Neurosurg. 2016;124:1531–7. [PubMed] [Google Scholar]
26. Thawani JP, Pisapia JM, Singh N, Petrov D, Schuster JM, Hurst RW, et al. 3D-Printed Modeling of an Arteriovenous Malformation Including Blood Flow. World Neurosurg. 2016;90:675–83. [PubMed] [Google Scholar]
27. Troebinger L, López JD, Lutti A, Bradbury D, Bestmann S, Barnes G. High precision anatomy for MEG. Neuroimage. 2014;86:583–91. [PMC free article] [PubMed] [Google Scholar]
28. Waran V, Pancharatnam D, Thambinayagam HC, Raman R, Rathinam AK, Balakrishnan YK, et al. The utilization of cranial models created using rapid prototyping techniques in the development of models for navigation training. J Neurol Surg A Cent Eur Neurosurg. 2013;75:12–5. [PubMed] [Google Scholar]
29. Waran V, Menon R, Pancharatnam D, Rathinam AK, Balakrishnan YK, Tung TS, et al. The creation and verification of cranial models using three-dimensional rapid prototyping technology in field of transnasal sphenoid endoscopy. Am J Rhinol Allergy. 2012;26:132–6. [PubMed] [Google Scholar]
30. Waran V, Narayanan V, Karuppiah R, Owen SL, Aziz T. Utility of multimaterial 3D printers in creating models with pathological entities to enhance the training experience of neurosurgeons. J Neurosurg. 2014;120:489–92. [PubMed] [Google Scholar]
31. Waran V, Narayanan V, Karuppiah R, Pancharatnam D, Chandran H, Raman R, et al. Injecting Realism in Surgical Training—Initial Simulation Experience With Custom 3D Models. J Surg Educ. 2014;71:193–7. [PubMed] [Google Scholar]
32. Waran V, Narayanan V, Karuppiah R, Thambynayagam HC, Muthusamy KA, Rahman ZAA, et al. Neurosurgical Endoscopic Training via a Realistic 3-Dimensional Model With Pathology. Simul Healthc. 2015;10:43–8. [PubMed] [Google Scholar]
33. Weinstock P, Prabhu SP, Flynn K, Orbach DB, Smith E. Optimizing cerebrovascular surgical and endovascular procedures in children via personalized 3D printing. Neurosurgery. 2015;16:1–6. [PubMed] [Google Scholar]
34. Whatley BR, Kuo J, Shuai C, Damon BJ, Wen X. Fabrication of a biomimetic elastic intervertebral disk scaffold using additive manufacturing. Biofabrication. 2011;3:015004. [PubMed] [Google Scholar]
35. Wurm G, Lehner M, Tomancok B, Kleiser R, Nussbaumer K. Cerebrovascular biomodeling for aneurysm surgery: Simulation-based training by means of rapid prototyping technologies. Surg Innov. 2011;18:294–306. [PubMed] [Google Scholar]
36. Wurm G, Tomancok B, Pogady P, Holl K, Trenkler J. Cerebrovascular stereolithographic biomodeling for aneurysm surgery. Technical note. J Neurosurg. 2004;100:139–45. [PubMed] [Google Scholar]
37. Xu WH, Liu J, Li ML, Sun ZY, Chen J, Wu JH. 3D printing of intracranial artery stenosis based on the source images of magnetic resonance angiograph. Ann Transl Med. 2014;2:74. [PMC free article] [PubMed] [Google Scholar]
3D printing in neurosurgery education: a review | 3D Printing in Medicine
- Review
- Open Access
- Published:
- Grace M. Thiong’o1,2,
- Mark Bernstein3 &
- James M.
Drake1,2
3D Printing in Medicine volume 7, Article number: 9 (2021) Cite this article
-
2606 Accesses
-
12 Citations
-
2 Altmetric
-
Metrics details
Abstract
Objectives
The objectives of this manuscript were to review the literature concerning 3D printing of brain and cranial vault pathology and use these data to define the gaps in global utilization of 3D printing technology for neurosurgical education.
Methods
Using specified criteria, literature searching was conducted to identify publications describing engineered neurosurgical simulators. Included in the study were manuscripts highlighting designs validated for neurosurgical skill transfer. Purely anatomical designs, lacking aspects of surgical simulation, were excluded. Eligible manuscripts were analyzed. Data on the types of simulators, representing the various modelled neurosurgical pathologies, were recorded. Authors’ countries of affiliation were also recorded.
Results
A total of thirty-six articles, representing ten countries in five continents were identified. Geographically, Africa as a continent was not represented in any of the publications. The simulation-modelling encompassed a variety of neurosurgical subspecialties including: vascular, skull base, ventriculoscopy / ventriculostomy, craniosynostosis, skull lesions / skull defects, intrinsic brain tumor and other. Finally, the vascular and skull base categories together accounted for over half (52.8 %) of the 3D printed simulated neurosurgical pathology.
Conclusions
Despite the growing body of literature supporting 3D printing in neurosurgical education, its full potential has not been maximized. Unexplored areas of 3D printing for neurosurgical simulation include models simulating the resection of intrinsic brain tumors or of epilepsy surgery lesions, as these require complex models to accurately simulate fine dissection techniques. 3D printed surgical phantoms offer an avenue for the advancement of global-surgery education initiatives.
Introduction
Rapid prototyping has seen a rise in application since its infancy in the 1980’s [1]. It can be used to personalize health care through the process of fabricating patient-specific models [2]. This technology, also referred to as three-dimensional (3D) printing, when coupled with high-fidelity specifications or haptic feedback has led to the simulation of various intra-operative scenarios [3]. These simulations have in turn produced feedback data useful for face, construct and content validity of many models in different individual studies [4,4,5,6,7,8,9]. Additionally, validation studies have proven the resulting phantom applications in surgical training to be reproducible as well as to act as reasonable alternatives to cadaveric dissection [10,11,12].
The rapid adaptation of 3D printing technology in surgical training results in part from the conflict created by an increasing demand of surgical skill proficiency within a work-hour restricted environment [13]. The resulting simulators are a reasonable supplement for surgical apprenticeship. The intricacies of the surgical practice have seen an incorporation of phantoms in various surgical residency training programs, including neurosurgery [3, 14]. In the Low and Middle Income Countries (LMIC), there is a mismatch between the large neurosurgical volume and the much fewer number of neurosurgeons. Dewan et al. highlighted the gross disparity in the allocation of surgical workforce, that left large geographic treatment gaps, particularly in Africa [15]. The training of more surgeons has been proffered as a strategy to bridge this disparity [16]. Training of neurosurgeons through the traditional method of apprenticeship is not without its disadvantages, such as patient risk. 3D printed simulators overcome this shortcoming by presenting opportunities to equip trainees with common neurosurgical techniques as well as introducing complex skill sets to qualified neurosurgeons prior to carrying out live surgeries.
The core neurosurgical training sub-specialties are neuro-oncology, pediatric, functional, neurovascular, neuro-trauma, skull base and spine surgery. The shortcomings of technical skill transfer for these areas of specialization arising from cadaveric training, such as and more recently through on 3D printed surgical simulators.
The aims of this manuscript are to review the current global innovations of 3D printing in neurosurgical training, to identify both its global geographic distribution as well as the gaps in technical skill acquisition that 3D printing technology can potentially fill.
Methods
A literature search was conducted on PubMed and Ovid for articles describing the design and application of 3D printing technology for neurosurgical training. The MeSH terms used were the following: “models / anatomic”, “neurosurgery education” and “printing, three dimensional”. The search concepts “models / anatomic”, “neurosurgery education” and “rapid prototyping” as well as “models / anatomic”, “neurosurgery education” and additive manufacturing” were also entered. PRISMA guidelines were applied for the identification, screening, eligibility checks and inclusion of relevant manuscripts [17]. Articles discussing phantoms of the brain, brainstem, intracranial vascular pathology and craniosynostosis were included. Those describing design and use of 3D printed surgical guides were likewise included, as were publications detailing approaches to the skull base. Manuscripts focusing purely on the neuroanatomy; those not tailored to a specific neurosurgical pathology, as well as those that lacked surgeon (or surgeon-in-training) task validation were excluded. Others discussing spine, and spinal cord phantoms, bioprinting, isolated virtual 3D, and non-human modelling were likewise excluded. Although spine surgery is an important part of neurosurgical training, its simulation focuses on skill sets that differ from those of brain and cranial vault neurosurgery.
Journals included in the final review were analyzed for demographic characteristics, trends and country affiliation of the authors. The texts were scrutinized to ensure that the countries where the phantoms were created and validated matched that of at least one of the authors. Once the final review process was completed, the extracted data were grouped using broad identifying terms: vascular, skull base, ventriculoscopy / ventriculostomy, craniosynostosis, skull lesions / skull defects, intrinsic brain tumor and other. Statistical analysis was performed using R (The R Foundation for Statistical Computing, Vienna, Austria), JMP Pro 14 (JMP Statistical Discovery™ from SAS, North Carolina, USA) and geolytics (Geolytics Pte Ltd, Singapore) software packages.
Results
A summary of our search findings is illustrated in Fig. 1. A total of 36 articles representing ten countries were identified. Publications spanned the years between 2014 and 2020. In 2014, only one neurosurgery simulation manuscript was published, according to the outcome of the search criteria. In subsequent years, seven task-defining simulation phantoms were 3D printed in 2015, four in 2016, six in 2017, nine in 2018, seven in 2019 and two in the first 3.5 months of the year 2020. Figure 2 summarizes these findings in tabulated format.
Fig. 1PRISMA search strategy summary
Full size image
Fig. 2A tabulated summary of the distribution of the 3D printed phantoms identified in literature, corresponding to the author’s country affiliations
Full size image
Broad classifying terms grouped the inventions into seven domains: vascular, skull base, ventriculoscopy / ventriculostomy, craniosynostosis, skull lesions / skull defects, intrinsic brain tumor and other. Of the 36 simulators in our review, vascular models accounted for 10 and skull base models accounted for nine. The two categories, vascular and skull base together, account for over half (52.8 %) of the inventions. Ventriculoscopy / ventriculostomy was simulated in seven of the 36 publications (19.4 %), craniosynostosis in four of the 36 (11.1 %), skull lesions/defects in two of the 36 (5.5 %). Only one manuscript (2.8 %) simulated brain tumor surgery, which in this case was a patient-specific low-grade glioma resection. Single publications were also identified for epilepsy surgery (stereoelectroencephalography), congenital malformations (Sturge weber), and a final model that simulated brain retraction.
Of the 10 countries represented as having contributed to the data by virtue of author affiliation, publications from the USA alone accounted for 47.2 % of the total (17/36). China accounted for 19.4 % (7/36) of the simulated designs, Japan 11.1 % (4/36), Brazil and Canada each 5.6 % (2/36) and Switzerland and Netherlands each 2. 8 % (1/36). Co-authored publications included Australia-USA, Turkey-Canada, Canada-Netherlands and USA-Taiwan; there was one article per collaborating author team. Finally, the resulting numeric data were used to generate a world geographic heat map (Fig. 3) as well as a graphical illustration of the categorical distribution of the simulated inventions by country/author affiliation (Fig. 4).
Geographic heat map illustrating the current distribution of 3D print technology’s use in neurosurgical education
Full size image
Fig. 4A graphical illustration of the disaggregated data by country of author’s affiliation
Full size image
Disaggregation of the data yielded Fig. 4, a graph that illustrates the distribution of the various simulated design categories per author’s country of affiliation. Specifically, of the top three simulated categories vascular designs are clustered within China and the USA, skull base designs are clustered within China, Japan and the USA whereas ventriculoscopy designs have a fairly even distribution.
Discussion
The key findings of this manuscript are the geographic depiction of 3D printing technology for neurosurgical education, the trends seen in the timeline between 2014 and 2019 and the simulated neurosurgical disease. By extension this highlights the areas that have as yet not been adequately simulated such as resective epilepsy surgery and brain tumor microdissection techniques.
The review found a general upward trend in the relevant publications, between the years 2014 to 2019, describing the design and neurosurgical application of skull and central nervous system (CNS) simulators. The projected inventions, therefore, for the year 2020 are expected to rise.
Phantoms simulating neurovascular and skull base surgical techniques are relatively common, according to literature. On the other hand, 3D printed models simulating resective epilepsy surgery and brain tumor microdissection techniques are not well described in the literature. [1819]. This finding could indicate that 3D printing technology has not yet evolved to creating models that can simulate fine dissection detail. Currently, there exist a variety of soft materials for direct 3D printing such as TissueMatrix™ that can be used to fabricate quite complex designs using state of the art PolyJet technology, however, the high cost of manufacturing is a limitation. Additionally, despite the high physical fidelity of the resulting designs even this softest material lacks the appropriate tactile feel that would be ideal for simulating neurosurgical dissection. Perhaps not so surprising are the findings in the geographic heat map (Fig. 3), illustrating the paucity of 3D printing technology in the continent of Africa. The call for surgeons to engage in education on a global platform has been made [20, 21]. Our article introduces one way that the global-surgery education gap can be met through the versatile use of 3D printed surgical simulators. Introducing reusable simulators such as those validated for ventriculoscopy is a cost-effective strategy to train resident neurosurgeons both in the developing as well as the developed world.
In higher income countries, where evolving surgical engineering research is feasible, developing new materials or re-inventing the use of existing materials which have higher neurosurgical functional fidelity could be explored.
In the developed world the clustering of inventions observed following disaggregation of our data (Fig. 4) is likely multifactorial. The divergence of biomedical engineering research to a surgical engineering focus would occur at different speeds within different institutions. In addition, it is possible that the observed clustering could be representative of the neuropathologic prevalence within each locality [22]. Consequently, the prevalence of disease would stimulate collaboration between surgeons and clinical engineers in the affected countries.
With regard to the representation of countries within North America all the Canadian publications meeting our inclusion criteria originated from the Center for Image Guided Innovation and Therapeutic Intervention lab (CIGITI) at The Hospital for Sick Children [5, 6, 23, 24].
Conclusions
Overall, our review article argues that the already-proven benefit of 3D printing technology for surgical training could be the next frontier in global-surgery education.
Availability of data and materials
All data generated or analyzed during this study are included in this published article.
References
Horvath J, Horvath JA. Brief History of 3D Printing. Mastering 3D Print. 2014. https://doi.org/10.1007/978-1-4842-0025-4_1.
Randazzo M, Pisapia J, Singh N, Thawani J. 3D printing in neurosurgery: A systematic review. Surg Neurol Int. 2016. doi:https://doi.org/10.4103/2152-7806.194059.
Article PubMed PubMed Central Google Scholar
Badash I, Burtt K, Solorzano CA, Carey JN. Innovations in surgery simulation: A review of past, current and future techniques.
Ann Transl Med. 2016. doi:https://doi.org/10.21037/atm.2016.12.24.
Article PubMed PubMed Central Google Scholar
Zhu J, Yang J, Tang C, Cong Z, Cai X, Ma C. Design and validation of a 3D-printed simulator for endoscopic third ventriculostomy. Child’s Nerv Syst. 2020. https://doi.org/10.1007/s00381-019-04421-8.
Article Google Scholar
Acar T, Karakas AB, Ozer MA, Koc AM, Govsa F. Building Three-Dimensional Intracranial Aneurysm Models from 3D-TOF MRA: a Validation Study. J Digit Imaging. 2019. https://doi.org/10.1007/s10278-019-00256-6.
Article PubMed PubMed Central Google Scholar
Bodani VP, Breimer GE, Haji FA, Looi T, Drake JM. Development and evaluation of a patient-specific surgical simulator for endoscopic colloid cyst resection.
J Neurosurg. 2019. https://doi.org/10.3171/2019.4.JNS183184.
Article PubMed Google Scholar
Grillo FW, Souza VH, Matsuda RH, Rondinoni C, Pavan TZ, Baffa O, et al. Patient-specific neurosurgical phantom: assessment of visual quality, accuracy, and scaling effects. 3D Print Med. 2018. https://doi.org/10.1186/s41205-018-0025-8.
Weinstock P, Prabhu SP, Flynn K, Orbach DB, Smith E. Optimizing cerebrovascular surgical and endovascular procedures in children via personalized 3D printing. J Neurosurg Pediatr. 2015. https://doi.org/10.3171/2015.3.PEDS14677.
Article PubMed Google Scholar
Yi Z, He B, Liu Y, Huang S, Hong W. Development and evaluation of a craniocerebral model with tactile-realistic feature and intracranial pressure for neurosurgical training. J Neurointerv Surg. 2020. https://doi.
org/10.1136/neurintsurg-2019-015008.
Article PubMed Google Scholar
Hsieh TY, Dedhia R, Cervenka B, Tollefson TT. 3D Printing: Current use in facial plastic and reconstructive surgery. Curr Opin Otolaryngol Head Neck Surg. 2017. https://doi.org/10.1097/MOO.0000000000000373.
Article PubMed Google Scholar
Rose AS, Kimbell JS, Webster CE, Harrysson OLA, Formeister EJ, Buchman CA. Multi-material 3D models for temporal bone surgical simulation. Ann Otol Rhinol Laryngol. 2015. https://doi.org/10.1177/0003489415570937.
Article PubMed Google Scholar
Hochman JB, Rhodes C, Wong D, Kraut J, Pisa J, Unger B. Comparison of cadaveric and isomorphic three-dimensional printed models in temporal bone education. Laryngoscope. 2015. https://doi.
org/10.1002/lary.24919.
Article PubMed Google Scholar
Hutter MM, Kellogg KC, Ferguson CM, Abbott WM, Warshaw AL. The impact of the 80-hour resident workweek on surgical residents and attending surgeons. Ann Surg. 2006. doi:https://doi.org/10.1097/01.sla.0000220042.48310.66.
Article PubMed PubMed Central Google Scholar
Klein GT, Lu Y, Wang MY. 3D printing and neurosurgery–ready for prime time? World Neurosurg. 2013. https://doi.org/10.1016/j.wneu.2013.07.009.
Article PubMed Google Scholar
Dewan MC, Rattani A, Fieggen G, Arraez MA, Servadei F, Boop FA, et al. Global neurosurgery: The current capacity and deficit in the provision of essential neurosurgical care. Executive summary of the global neurosurgery initiative at the program in global surgery and social change.
J Neurosurg. 2019. https://doi.org/10.3171/2017.11.JNS171500.
Article PubMed Google Scholar
Park KB, Johnson WD, Dempsey RJ. Global Neurosurgery: The Unmet Need. World Neurosurg. 2016. https://doi.org/10.1016/j.wneu.2015.12.048.
Moher D, Liberati A, Tetzlaff J, Altman DG, Group TP. Preferred Reporting Items for Systematic Reviews and Meta-Analyses: The PRISMA Statement. PLoS Med [Internet]. Public Library of Science; 2009. https://doi.org/10.1093/ptj/89.9.873.
Thawani JP, Singh N, Pisapia JM, Abdullah KG, Parker D, Pukenas BA, et al. Three-dimensional printed modeling of diffuse low-grade gliomas and associated white matter tract anatomy. Clin Neurosurg. 2017. https://doi.org/10.1093/neuros/nyx009.
Article Google Scholar
Camara D, Panov F, Oemke H, Ghatan S, Costa A.
Robotic surgical rehearsal on patient-specific 3D-printed skull models for stereoelectroencephalography (SEEG). Int J Comput Assist Radiol Surg. 2019. https://doi.org/10.1007/s11548-018-1885-5.
Article PubMed PubMed Central Google Scholar
Meara JG, Greenberg SLM. The Lancet Commission on global surgery global surgery 2030: Evidence and solutions for achieving health, welfare and economic development. Surg (United States). 2015. doi:https://doi.org/10.1016/j.surg.2015.02.009.
Article Google Scholar
Farmer PE, Kim JY. Surgery and global health: A view from beyond the OR. World J Surg. 2008. doi:https://doi.org/10.1007/s00268-008-9525-9.
Article PubMed PubMed Central Google Scholar
Wu S, Wu B, Liu M, Chen Z, Wang W, Anderson CS, et al.
Stroke in China: advances and challenges in epidemiology, prevention, and management. Lancet Neurol. 2019. https://doi.org/10.1016/S1474-4422(18)30500-3.
Article PubMed Google Scholar
Eastwood KW, Bodani VP, Haji FA, Looi T, Naguib HE, Drake JM. Development of synthetic simulators for endoscope-assisted repair of metopic and sagittal craniosynostosis. J Neurosurg Pediatr. 2018. https://doi.org/10.3171/2018.2.PEDS18121.
Article PubMed Google Scholar
Breimer GE, Bodani V, Looi T, Drake JM. Design and evaluation of a new synthetic brain simulator for endoscopic third ventriculostomy. J Neurosurg Pediatr. 2015. https://doi.org/10.3171/2014.9.PEDS1447.
Article PubMed Google Scholar
Download references
Acknowledgements
Dr. Rachael Cayley PhD, Associate Professor at the School of Graduate Studies, University of Toronto, helped to grammar-proof our article.
Funding
None.
Author information
Authors and Affiliations
Center for Image Guided Innovation and Therapeutic Intervention, Toronto, Canada
Grace M. Thiong’o & James M. Drake
Division of Neurosurgery, Hospital for Sick Children, University of Toronto, 555 University Avenue, Ontario, M5G 1X8, Toronto, Canada
Grace M. Thiong’o & James M. Drake
Division of Neurosurgery, Toronto Western Hospital, University of Toronto, Ontario, Toronto, Canada
Mark Bernstein
Authors
- Grace M. Thiong’o
View author publications
You can also search for this author in PubMed Google Scholar
- Mark Bernstein
View author publications
You can also search for this author in PubMed Google Scholar
- James M. Drake
View author publications
You can also search for this author in PubMed Google Scholar
Contributions
Conception and design: Thiong’o. Acquisition of data: Thiong’o. Analysis and interpretation of data: Thiong’o. Drafting the article: All authors. Critically revising the article: All authors. Reviewed submitted version of manuscript: All authors. Approved the final version of the manuscript on behalf of all authors: Thiong’o. Statistical analysis: Thiong’o. Study supervision: Drake.
Corresponding author
Correspondence to Grace M. Thiong’o.
Ethics declarations
Authors’ Information
All the authors are committed to finding innovative solutions to the global neurosurgery inequities.
Ethics approval and consent to participate
Not applicable.
Consent for Publication
Not applicable.
Competing interests
The authors declare that they have no competing interests.
Additional information
Publisher’s Note
Springer Nature remains neutral with regard to jurisdictional claims in published maps and institutional affiliations.
Rights and permissions
Open Access This article is licensed under a Creative Commons Attribution 4.0 International License, which permits use, sharing, adaptation, distribution and reproduction in any medium or format, as long as you give appropriate credit to the original author(s) and the source, provide a link to the Creative Commons licence, and indicate if changes were made. The images or other third party material in this article are included in the article's Creative Commons licence, unless indicated otherwise in a credit line to the material. If material is not included in the article's Creative Commons licence and your intended use is not permitted by statutory regulation or exceeds the permitted use, you will need to obtain permission directly from the copyright holder. To view a copy of this licence, visit http://creativecommons.org/licenses/by/4.0/. The Creative Commons Public Domain Dedication waiver (http://creativecommons.org/publicdomain/zero/1.0/) applies to the data made available in this article, unless otherwise stated in a credit line to the data.
Reprints and Permissions
About this article
Application of 3D printing in pediatric neurosurgery.
Introduction.
I am a neurosurgeon in one of the leading national centers in the country. For a long time I wanted to share my experience in applying 3D printing in everyday work. And I consider the main goal of my article to show the possibilities and reality of using printing in medicine. The site has a large number of articles about the printing of organs, bones, implants, but the vast majority of them are episodic cases, descriptions of foreign developments or over-hyped records based on articles from the media that do not delve into the essence of the problem. I will try to briefly describe my experience in using 3D printing in clinical practice. nine0005
Science.
I started my journey in 3D printing by analyzing the literature. If you type in the query '3d printing' at www.ncbi.nlm.nih. gov, you can see a funny curve in the number of publications each year.
In terms of areas of application, for 2017 the data is approximately as follows:
Surgical recommendations - 60%
Model making for surgical planning - 38.7%
Custom implants - 12.1% (titanium, PEEK, hydroxyapatite , methacrylate, silicone)
Orthoses and prostheses - 3.9%
Pattern - 1.7%
Selection of patients for surgery - 0.8%
Applications:
MFS - 24% %
Spinal surgery - 7.4%
Vascular neurosurgery - 1.3%
It should be noted that there are practically no solid publications on 3D printing in Russia. I looked through all the publications on demand in elibrary.ru, and was somewhat disappointed. This is due, on the one hand, to the fact that in our country there are no certified methods, printers and materials for printing and use, on the other hand, doctors do not actually receive significant profit from the technology, taking into account personal and financial costs. For the most part, all the cases described on the site are the enthusiasm of individuals, less often - student circles. nine0005
What can you do?
It seems that dental models and implants and insoles have the greatest practical use, but here colleagues can correct me.
As for neurosurgery, 3D SLS models made of titanium for cranioplasty (for example, such models are made at N.N. Burdenko National Medical Research Center) and biopolymer models based on PEEK and PMMA (the experience of using them available at the Research Institute of Emergency Pediatric Surgery and Traumatology). The volume of operations performed using such technologies per year will not exceed a couple of dozen. nine0005
A more promising area of application is the production of models for preoperative planning. Traumatology and neurosurgery take the first place here. It is on this part that I will dwell in more detail later. It should be noted that the production of prototypes for preparation for the operation does not require special certification.
Also, printing of demonstration models for students, residents and patients themselves is quite an extensive area, since the visual presentation makes it much easier to communicate with a person who is far from human anatomy. nine0005
Model creation.
I won't retell the widely covered techniques for importing STL from DICOM, I'll focus only on a couple of features that seemed important to me.
For almost all models, I used InVesalius, because its interface and model creation speed favorably distinguish it from the same 3DSlicer. I would like to immediately warn enthusiasts who want to print anatomical models of organs - it will come out dumb. Briefly for the layman: computed tomography is a method based on the absorption of x-rays by tissues. It well differentiates tissues by density: air is black, soft tissues are gray, bone is black. nine0005
Tumors, vessels, parenchymal organs will not be visible. InVesalius will see a density difference of a couple of hundred Housefield Units (HU), and all organs have a difference of 15-30 HU. The only exception will be a contrast study of the vessels, and then only if the time of passage of the contrast is correctly selected, otherwise the difference with soft tissues will be minimal.
As for magnetic resonance imaging - it is based on the resonance of hydrogen atoms, tissues with different concentrations of water / proteins / fats / metals are well distinguished. nine0005
It is almost impossible to get a 3D model from such a sequence of images, or post-processing will take a catastrophic amount of time. The same applies to tumors with a pronounced vascular network - at the level of medium-sized vessels, a lot of disparate objects will turn out, it is unrealistic to print this. I do not consider it necessary to print the main vessels in preoperative preparation - the surgeon most often knows where the line passes.
Thus, it is possible to print bone density formations (actually bones, calcifications, foreign bodies - bullets, blades, shot . ..) and contrast studies (vessels, heart chambers) at low cost. nine0005
Preoperative preparation.
At the moment my material is 18 models. All models are made according to the data of computed tomography of patients aged from 1 month to 14 years. Of these, there are 13 skull models, 3 vessels models, 2 spine models.
Models were printed on 2 printers: Ultimaker 2 and Designer X PRO.
8 models were used in preoperative planning. The essence of the pathology - craniosynostosis - is a congenital deformity of the skull associated with the early closure of cranial sutures. Children with such a pathology need to undergo reconstructive surgery to correct the shape of the skull. After receiving the finished model, removal of supports and post-processing, an 'osteotomy' was performed using a mini-drill - the skull was sawn as it is supposed to be during the operation. The finished fragments were remodeled, placed in the correct position and fixed with titanium plates, which were also adjusted during planning. In some cases, these plates were sent for sterilization and implanted in the patient. nine0005
Clinical example.
Child with Crouzon syndrome, bicoronary craniosynostosis. It was previously operated on in another institution. He was admitted with complaints of extensive defects in the bones of the skull with prolapse of the medulla into bone defects. An additional examination revealed craniocerebral disproportion - a discrepancy between the volume of the skull and the volume of the brain. The child needed to simultaneously perform expansion reconstruction and defect cranioplasty. A model of the skull was made, 1:1 in size. nine0005
Rendering DICOM images:
And the model itself after printing and removal of supports (PLA). This particular model was printed using Ultimaker 2.
Due to the need for reconstruction of the upper orbits, expansion of the frontal parts of the skull, extensive defects of the vault were formed. Even before the operation, osteotomy lines were outlined on the template, and a titanium mesh was modeled to close the defect. The operation itself took 7 hours, which was associated with severe cicatricial adhesions after the first operation. The final version on computed tomography looked like this:
Another 7 patients were planned in this way. The time period for this work is 1 year. I did not try to make models for all planned operations - this is not necessary. But in complex clinical cases, initially having an idea of the anatomical structure is priceless.
The remaining models were used as demonstrations and training models, which also made a lot of sense. Having become interested in this topic, we even managed to find evidence-based studies confirming the improvement in the assimilation of the material when using various material anatomical models. nine0005
Conclusions/Conclusion.
At the present stage of development of medicine, the use of 3D models as implants remains a rare phenomenon. Unfortunately, there is no big work in the area. As for the production of layouts for planning - the area has both development prospects and real practical value, at least this helped my patient.
I would like to express my deep gratitude to the people who helped with advice and printing of all models:
Ivan Nikolaevich Moskvichev , known as plastmaska - the realization of the idea would have been impossible without his help;
Andrey Viktorovich Isupov, Vato - for technical support, assistance in printing models;
Kucherova Polina Alekseevna - for informational and technical support.
If you are interested in some specific details on the use of models, maybe something in processing or getting the STL itself - I will try to answer, write in the comments. Finally, just a few more photos. nine0005
3D printing technologies helped St. Petersburg neurosurgeons perform a complex operation
News
and 3D printing in planning a surgical operation to remove a tumor in the eye socket of the skull of a teenage patient.
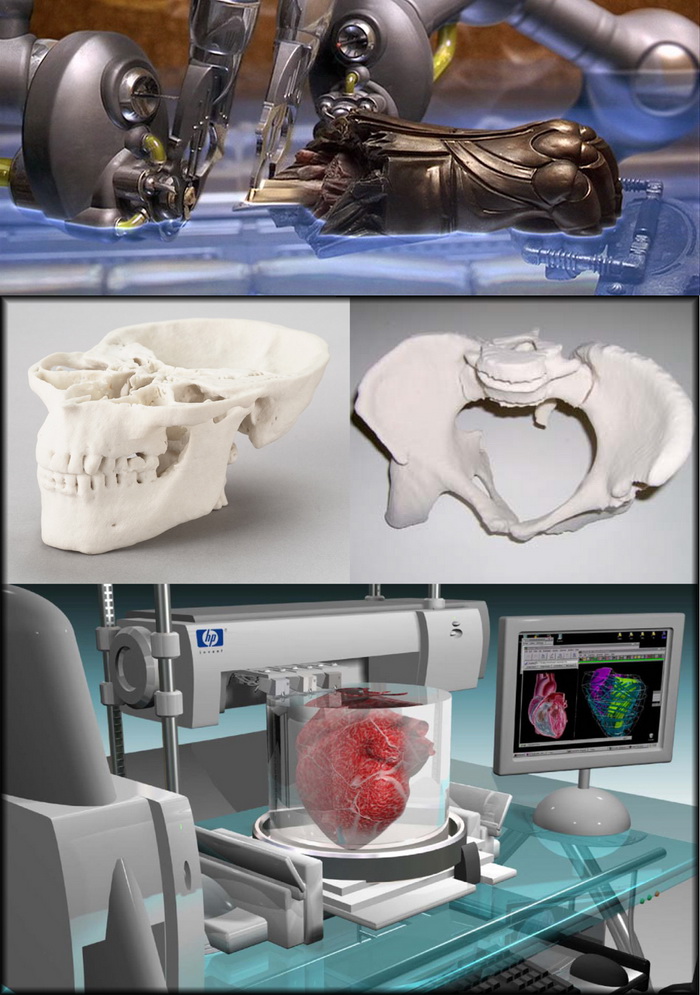
According to the press service of the Almazov Center, 3D printing technologies have been used by the staff of the institution since 2017 when planning surgical interventions in children with complex concomitant malformations of the skull, tumors of the skull bones and trepanation defects of a complex configuration. Additive technologies make it possible to model implants taking into account individual anatomy, and in cases with tumors of the skull base and orbit, to plan in advance the trajectory of the surgical approach and the minimum sufficient volume of craniotomy, which significantly reduces the invasiveness of operations, as well as improves functional and cosmetic results. nine0005
Vadim Ivanov (right) and colleagues prepare for surgery
3D models of operated areas are created from computed tomography data, after which plastic layouts are printed to help doctors plan the stages of the operation and create surgical templates. At the Almazov Center, 3D printing technologies are being introduced at the initiative of pediatric neurosurgeon Vadim Ivanov. The Russian manufacturer of 3D printers PICASO 3D helped with the technical side of the matter: before Vadim mastered 3D printing technologies himself, the company assisted with medical prototyping on Designer X PRO branded 3D printers. nine0005
At the end of 2020, a teenage patient came to the Almazov Center complaining of swelling of the eyeball (exophthalmos) and pain in the left superciliary region. Examination revealed a volumetric formation of the left orbit, penetrating into the walls of the orbit with spread into the cranial cavity. Doctors performed a minimally invasive operation to remove a tumor at the base of the skull. The operation lasted an hour and a half, during which time the specialists performed an osteoplastic resection of the zygomatic process of the frontal bone, removed the mass and affected structures. The bone flap was subsequently placed in its original position with fixation with biodegradable miniplates. The superimposed cosmetic seam will make the scar from the operation invisible. nine0005
Left: 3D reconstruction of the skull before surgery. Volumetric formation destroys the roof of the orbit, penetrates into the zygomatic process of the frontal bone. Right: Preoperative 3D modeling. Volumetric education is highlighted in pink. Illustrations: Almazov Center
“Using modern methods of neuroimaging, followed by the construction of a virtual model, a real-size 3D model of the tumor with its surrounding structures was created. Such thorough preoperative preparation with planning the trajectory of surgical actions, lines of bone dissection and the least sufficient degree of resection of invasive structures made it possible to perform the operation through a minimal access,” said Vadim Ivanov. nine0005
Since 2017, more than fifty operations have been performed at the Almazov Center using additive technologies for various neurosurgical pathologies. In 2020, the Ministry of Health of the Russian Federation adopted for implementation a clinical testing protocol developed by specialists from the pediatric neurosurgical department, “Clinical testing of a method for preoperative planning of cranial reconstruction using 3D printing in children with complex synostotic deformities of the skull for preoperative preparation in comparison with the use of stereolithographic models.