3D printing aortic valve
Engineers 3D print lifelike heart valve models
Every year thousands of Americans, mostly over age 75, require replacement of their aortic valve. Now 3D printed patient-specific models of the aorta can aid presurgical planning and improve outcomes of minimally invasive valve replacement.
3D-printed model of a patient’s aortic region. The patient-specific model includes the anatomy of the damaged leaflets of the aortic valve, where the replacement valve will be inserted. The model includes integrated sensors that helps to guide positioning of the replacement valve. Credit: Haghiashtiani, et al. Science Advances, 28 Aug 2020 (ref. 2)The left ventricle of the heart has the important function of pushing fresh oxygenated blood through to the aorta, which then distributes blood throughout the body. The aortic valve opens as the blood is pushed through and then closes so blood cannot move backwards into the heart. Aortic stenosis is a condition where the aortic valve has become narrow over time, causing the heart to work harder—a condition that can lead to heart failure.
Transcatheter aortic valve replacement (TAVR) is a procedure where an artificial heart valve is threaded with a catheter through the aorta and expanded—much like opening an umbrella—to replace a failing aortic valve. TAVR is increasingly being used because it is a minimally invasive procedure that is much safer than open heart surgery for the predominantly elderly patients who require the procedure. However, because of irregularities of the diseased valve and patient-specific contours of the aorta, getting a perfect leak-proof fit and optimal positioning of the new valve is not always assured.
Now NIBIB-funded engineers at the University of Minnesota, led by Michael McAlpine, who holds the Kuhrmeyer Family Chair Professorship in the University of Minnesota Department of Mechanical Engineering, have made significant inroads toward improving TAVR by 3D printing a precise model of a patient’s diseased valve and surrounding parts of the aorta. This physical model of the region—known as the aortic root—allows physicians to practice the procedure needed for each patient, allowing them to identify the right size replacement valve and determine the exact placement in the aorta that will give the best fit and function.
“As the US population ages, the number of individuals over 75 needing valve replacement using TAVR will continue to grow,” explained Michael Wolfson, director of the NIBIB program in bionic systems. “The ability to create patient-specific models of the aortic root is a major development that could improve the success rate of this challenging procedure and significantly reduce the risks of postoperative complications.”
Although aortic root models have been used before for surgical planning, the models created by the advanced 3D printing technique are much more lifelike in texture and elasticity. Another unique aspect of these models is the incorporation of sensors in the wall of the aorta. The sensors provide feedback that allows physicians to avoid the application of excessive pressure by the replacement valve to the conduction pathway that controls heart rhythm. Too much pressure can cause abnormal heart rhythms and the immediate need to implant a permanent pacemaker in the patient.
The engineering team uses CT scans of the patient’s heart to recreate the exact shape of the aortic root region. They are then 3D printed with specialized silicone-based inks that match the feel of real heart tissue. The soft electric sensors that give pressure feedback are also 3D printed into the model and are made of a conducting hydrogel.
"This 3D-printing process was designed to help physicians improve the outcomes of the TAVR procedure and can also help give patients a clearer understanding of their anatomy and how the procedure works” said McAlpine. As engineers continue to improve 3D printing techniques and create even more lifelike models of organs, McAlpine envisions where such technologies could lead. “In the future, as our 3D-printed models incorporate more aspects of organ function, we see the potential for the models themselves to someday be used as artificial replacement organs. "
The work was reported in the journal Science Advances1. Funding was provided via grants from the National Institute of Biomedical Imaging and Bioengineering (DP2EB020537), Medtronic plc, and the MnDRIVE program at the University of Minnesota.
1. 3D printed patient-specific aortic root models with internal sensors for minimally invasive applications. Haghiashtiani G, Qiu K, Zhingre Sanchez JD, Fuenning ZJ, Nair P, Ahlberg SE, Iaizzo PA, McAlpine MC. Sci Adv. 2020 Aug 28;6(35):eabb4641. doi: 10.1126/sciadv.abb464
2. https://creativecommons.org/licenses/by-nc/4.0/
Researchers 3D print lifelike heart valve models
Research Brief
Patient-specific organ models, which include integrated 3D-printed soft sensor arrays, are fabricated using specialized inks and a customized 3D printing process. Such models can be used in preparation for minimally invasive procedures to improve outcomes in thousands of patients worldwide.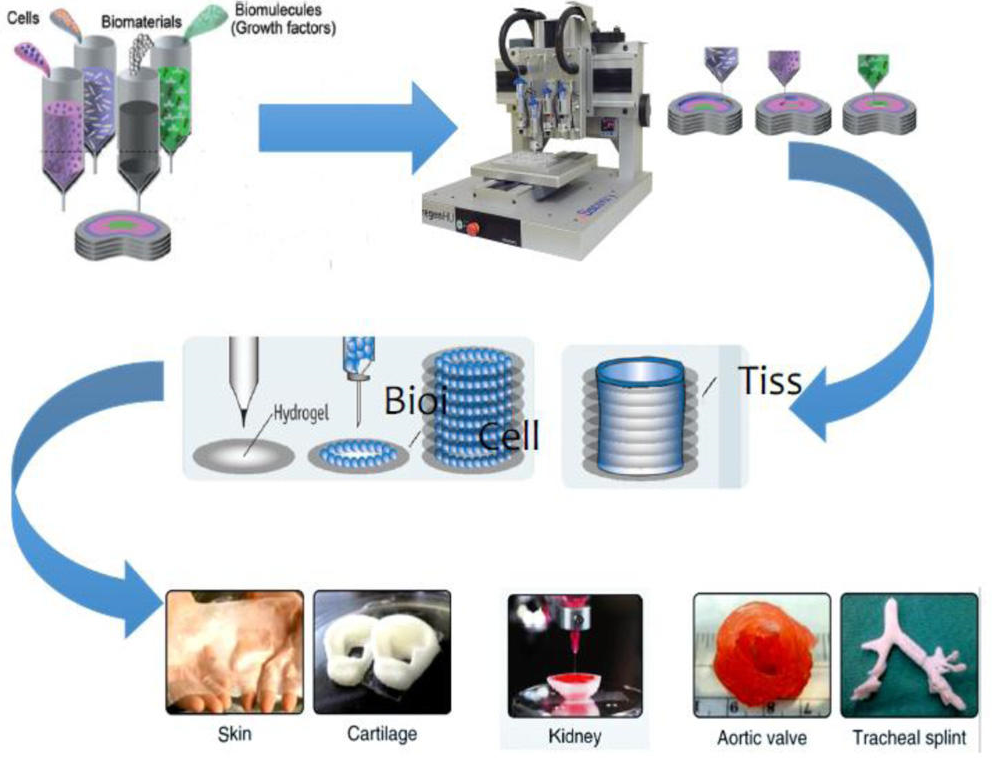
Researchers from the University of Minnesota, with support from Medtronic, have developed a groundbreaking process for multi-material 3D printing of lifelike models of the heart’s aortic valve and the surrounding structures that mimic the exact look and feel of a real patient.
These patient-specific organ models, which include 3D-printed soft sensor arrays integrated into the structure, are fabricated using specialized inks and a customized 3D printing process. Such models can be used in preparation for minimally invasive procedures to improve outcomes in thousands of patients worldwide.
The research is published in Science Advances, a peer-reviewed scientific journal published by the American Association for the Advancement of Science (AAAS).
The researchers 3D printed what is called the aortic root, the section of the aorta closest to and attached to the heart. The aortic root consists of the aortic valve and the openings for the coronary arteries. The aortic valve has three flaps, called leaflets, surrounded by a fibrous ring. The model also included part of the left ventricle muscle and the ascending aorta.
“Our goal with these 3D-printed models is to reduce medical risks and complications by providing patient-specific tools to help doctors understand the exact anatomical structure and mechanical properties of the specific patient’s heart,” said Michael McAlpine, a University of Minnesota mechanical engineering professor and senior researcher on the study. “Physicians can test and try the valve implants before the actual procedure. The models can also help patients better understand their own anatomy and the procedure itself.”
This organ model was specifically designed to help doctors prepare for a procedure called a Transcatheter Aortic Valve Replacement (TAVR) in which a new valve is placed inside the patient’s native aortic valve. The procedure is used to treat a condition called aortic stenosis that occurs when the heart’s aortic valve narrows and prevents the valve from opening fully, which reduces or blocks blood flow from the heart into the main artery. Aortic stenosis is one of the most common cardiovascular conditions in the elderly and affects about 2.7 million adults over the age of 75 in North America. The TAVR procedure is less invasive than open heart surgery to repair the damaged valve.
The aortic root models are made by using CT scans of the patient to match the exact shape. They are then 3D printed using specialized silicone-based inks that mechanically match the feel of real heart tissue the researchers obtained from the University of Minnesota’s Visible Heart Laboratories. Commercial printers currently on the market can 3D print the shape, but use inks that are often too rigid to match the softness of real heart tissue.
On the flip side, the specialized 3D printers at the University of Minnesota were able to mimic both the soft tissue components of the model, as well as the hard calcification on the valve flaps by printing an ink similar to spackling paste used in construction to repair drywall and plaster.
Physicians can use the models to determine the size and placement of the valve device during the procedure. Integrated sensors that are 3D printed within the model give physicians the electronic pressure feedback that can be used to guide and optimize the selection and positioning of the valve within the patient’s anatomy.
But McAlpine doesn’t see this as the end of the road for these 3D-printed models.
“As our 3D-printing techniques continue to improve and we discover new ways to integrate electronics to mimic organ function, the models themselves may be used as artificial replacement organs,” said McAlpine, who holds the Kuhrmeyer Family Chair Professorship in the University of Minnesota Department of Mechanical Engineering. “Someday maybe these ‘bionic’ organs can be as good as or better than their biological counterparts.”
In addition to McAlpine, the team included University of Minnesota researchers Ghazaleh Haghiashtiani, co-first author and a recent mechanical engineering Ph. D. graduate who now works at Seagate; Kaiyan Qiu, another co-first author and a former mechanical engineering postdoctoral researcher who is now an assistant professor at Washington State University; Jorge D. Zhingre Sanchez, a former biomedical engineering Ph.D. student who worked in the University of Minnesota’s Visible Heart Laboratories who is now a senior R&D engineer at Medtronic; Zachary J. Fuenning, a mechanical engineering graduate student; Paul A. Iaizzo, a professor of surgery in the Medical School and founding director of the U of M Visible Heart Laboratories; Priya Nair, senior scientist at Medtronic; and Sarah E. Ahlberg, director of research & technology at Medtronic.
This research was funded by Medtronic, the National Institute of Biomedical Imaging and Bioengineering of the National Institutes of Health, and the Minnesota Discovery, Research, and InnoVation Economy (MnDRIVE) Initiative through the State of Minnesota. Additional support was provided by University of Minnesota Interdisciplinary Doctoral Fellowship and Doctoral Dissertation Fellowship awarded to Ghazaleh Haghiashtiani.
To read the full research paper, entitled “3D printed patient-specific aortic root models with internal sensors for minimally invasive applications,” visit the Science Advances website.
- Categories:
- Science and Technology
3D printing makes it possible to create aortic heart valves
3D printing makes it possible to create aortic heart valves
Tens of thousands of patients are diagnosed with heart valve disease every year, and many need life-saving surgery.
Scientists from the Imaging Department of the Piedmont Heart Institute, in collaboration with scientists from the Georgia Institute of Technology, developed a new manufacturing technique using the 3D printer heart valves that will match patient-specific valves that aim to improve pre-surgical planning processes for transateter aortic valve replacement (TAVR) surgery.
When a person is diagnosed with heart valve disease, and especially if they are at high risk for complications with open heart failure, they are forced to undergo transateter aortic valve replacement, which involves implanting a prosthetic valve in the heart to replace the damaged valve.
The new valves will be able to mimic the physiological properties of real parts of the heart, which will significantly increase the efficiency of transcatheter aortic valve replacement and avoid the complication known as paravalvular leakage (blood flow from the aorta back into the ventricle), which is a decisive factor in the life expectancy of a patient after transplantation.
In addition, 3D models of the valves will allow physicians to evaluate how well the prosthesis will fit to the aorta. Already, models created from CT of the patient's heart are fully consistent with the real organ and reliably predict the likelihood of blood leakage through a loose valve.
This means that the ability to predict PVL could allow healthcare professionals to better tailor a prosthetic valve to a particular patient and ultimately improve their recovery from TAVR.
A key part of this platform is a realistic, patient-specific 3D printed model of a heart valve that can help professionals choose the right prosthesis for better TAVR results. Prosthetic valves are available in a wide range of types and sizes from different manufacturers, but one of the most important factors for a positive outcome is that the patient's natural heart valve matches the type and size of the prosthesis as closely as possible. This is where 9 comes into play.0003 3D model .
The prosthetic heart valves were fabricated using Stratasys' PolyJet 3D multilayer printing technology, which allowed the research team to use a range of different materials to best mimic the varying textures and hardnesses of the human heart and its aortic tissue.
The printed model is able to tell what the valve leak will be and where it is even before the operation, this is a good indicator for understanding the possible causes of death in the short and long term.
Importantly, the development of metamaterials and multi-layer 3D printing of allowed researchers to closely mimic the mechanical behavior of natural heart valves, including the stress-stiffness behavior in soft tissues caused by elastin and collagen proteins.
Selecting the correct type and size and achieving the proper seal between the prosthesis and the natural heart valve wall is key to preventing blood leakage around the prosthesis. This is where a unique 3D model can help.
Models are created using a machine capable of printing 3D models from multi-material materials. Researchers can adjust design parameters such as bend diameter and length using the metamaterial used for printing, allowing them to more closely mimic the physiological properties of tissue.
For example, models can recreate conditions such as calcium precipitation, which is a major factor in aortic stenosis.
In addition, scientists are experimenting with embedding sensors in models, using a machine that can print nanomaterials on the valve wall according to the scheme. Sensors could potentially be used to monitor how much a prosthetic valve deforms the model. With these sensors, the printed heart valve can also be used as a template for preoperative monitoring.
The team believes its new prosthetic valve testing process can help improve TAVR operations and reduce the risk of leaks. Once the process is improved, it can be implemented in hospitals, helping physicians treat patients with greater accuracy
In addition, as technologies and materials for 3D printing continue to evolve, the team says that many existing barriers to the production of 3D printed medical models and devices will be overcome, allowing 3D printing on the cardiovascular system to become "an important everyday clinical tool for improving the patient's condition."
3D printed regenerative heart valves that grow with patients
Researchers at the Technical University of Munich and the University of Western Australia are using 3D printing technology to develop artificial heart valves made from a patient's own cells that grow as his aging.
This approach overcomes the shortcomings of conventional prosthetic heart valves, which last only a limited number of years and therefore require multiple replacement surgeries.
Scientists used a melt electroforming 3D printing process to create a porous scaffold* from a patient's own cells. Melt electrospinning is an advanced additive manufacturing technology for depositing predetermined micrometric fibers that works by combining an applied electric field, temperature and pressure to create a charged jet of molten polymer.
Researchers have used this technology to apply microfibers less than one-tenth the thickness of a human hair with extreme precision in a predetermined pattern, giving the resulting fiber scaffolds the desired characteristics.
Melt electrospinning* provides significant advantages over other fiber forming methods such as conventional electrospinning as it allows the fabrication of scaffolds with customized mechanical properties, macroporosity and pattern for a wide range of applications including implant tissue engineering and disease modeling.
Currently, if damaged heart valves cannot be repaired, current treatments include the implantation of a prosthetic valve, which should ideally remain in the patient's body for the rest of their lives. However, the service life of such valves is limited, so patients have to undergo numerous surgical interventions to replace them. This problem is especially relevant for pediatric patients, as they require new valves as their bodies grow.
The research team's regenerative approach to tissue engineering of the heart valve aims to overcome the limitations of existing mechanical and biological prostheses by making a valve that can grow and change with the patient. To do this, it was necessary to print a scaffold with sufficient porosity so that the cells could penetrate the structure and develop.
The team used a melt-blown 3D printer to create heart valve implants that mimic various tissue structures in a patient's own aortic heart valve. The digital platform printed complex patterned structures, which were then connected to tubular microporous hydrogel scaffolds.
The resulting printed structure was able to withstand the complex functions of a heart valve while remaining porous enough for the patient's own cells to colonize and proliferate.