3D printing and stem cells
3D bioprinting using stem cells
Review
. 2018 Jan;83(1-2):223-231.
doi: 10.1038/pr.2017.252. Epub 2017 Nov 1.
Chin Siang Ong 1 , Pooja Yesantharao 1 , Chen Yu Huang 2 , Gunnar Mattson 1 , Joseph Boktor 1 , Takuma Fukunishi 1 , Huaitao Zhang 1 , Narutoshi Hibino 1
Affiliations
Affiliations
- 1 Division of Cardiac Surgery, Johns Hopkins Hospital, Baltimore, MD.
- 2 Division of Cardiology, Johns Hopkins Hospital, Baltimore, MD.
- PMID: 28985202
- DOI: 10.1038/pr.2017.252
Review
Chin Siang Ong et al. Pediatr Res. 2018 Jan.
. 2018 Jan;83(1-2):223-231.
doi: 10.1038/pr.2017.252. Epub 2017 Nov 1.
Authors
Chin Siang Ong 1 , Pooja Yesantharao 1 , Chen Yu Huang 2 , Gunnar Mattson 1 , Joseph Boktor 1 , Takuma Fukunishi 1 , Huaitao Zhang 1 , Narutoshi Hibino 1
Affiliations
- 1 Division of Cardiac Surgery, Johns Hopkins Hospital, Baltimore, MD.
- 2 Division of Cardiology, Johns Hopkins Hospital, Baltimore, MD.
- PMID: 28985202
- DOI: 10.1038/pr.2017.252
Abstract
Recent advances have allowed for three-dimensional (3D) printing technologies to be applied to biocompatible materials, cells and supporting components, creating a field of 3D bioprinting that holds great promise for artificial organ printing and regenerative medicine. At the same time, stem cells, such as human induced pluripotent stem cells, have driven a paradigm shift in tissue regeneration and the modeling of human disease, and represent an unlimited cell source for tissue regeneration and the study of human disease. The ability to reprogram patient-specific cells holds the promise of an enhanced understanding of disease mechanisms and phenotypic variability. 3D bioprinting has been successfully performed using multiple stem cell types of different lineages and potency. The type of 3D bioprinting employed ranged from microextrusion bioprinting, inkjet bioprinting, laser-assisted bioprinting, to newer technologies such as scaffold-free spheroid-based bioprinting. This review discusses the current advances, applications, limitations and future of 3D bioprinting using stem cells, by organ systems.
Similar articles
-
Advancing Frontiers in Bone Bioprinting.
Ashammakhi N, Hasan A, Kaarela O, Byambaa B, Sheikhi A, Gaharwar AK, Khademhosseini A. Ashammakhi N, et al. Adv Healthc Mater. 2019 Apr;8(7):e1801048. doi: 10.1002/adhm.201801048. Epub 2019 Feb 8. Adv Healthc Mater.
2019. PMID: 30734530 Review.
-
Three-dimensional bioprinting of stem-cell derived tissues for human regenerative medicine.
Skeldon G, Lucendo-Villarin B, Shu W. Skeldon G, et al. Philos Trans R Soc Lond B Biol Sci. 2018 Jul 5;373(1750):20170224. doi: 10.1098/rstb.2017.0224. Philos Trans R Soc Lond B Biol Sci. 2018. PMID: 29786559 Free PMC article. Review.
-
Recent advances in bioprinting techniques: approaches, applications and future prospects.
Li J, Chen M, Fan X, Zhou H. Li J, et al. J Transl Med. 2016 Sep 20;14:271. doi: 10.1186/s12967-016-1028-0. J Transl Med. 2016. PMID: 27645770 Free PMC article. Review.
-
Tissue Engineering Applications of Three-Dimensional Bioprinting.
Zhang X, Zhang Y. Zhang X, et al. Cell Biochem Biophys. 2015 Jul;72(3):777-82. doi: 10.1007/s12013-015-0531-x. Cell Biochem Biophys. 2015. PMID: 25663505 Review.
-
Three-dimensional bioprinting in tissue engineering and regenerative medicine.
Gao G, Cui X. Gao G, et al. Biotechnol Lett. 2016 Feb;38(2):203-11. doi: 10.1007/s10529-015-1975-1. Epub 2015 Oct 14. Biotechnol Lett. 2016. PMID: 26466597 Review.
See all similar articles
Cited by
-
Constructing biomimetic liver models through biomaterials and vasculature engineering.
Lv W, Zhou H, Aazmi A, Yu M, Xu X, Yang H, Huang YYS, Ma L. Lv W, et al. Regen Biomater. 2022 Oct 12;9:rbac079.
doi: 10.1093/rb/rbac079. eCollection 2022. Regen Biomater. 2022. PMID: 36338176 Free PMC article. Review.
-
3D bioprinted mesenchymal stromal cells in skin wound repair.
Luo Y, Xu X, Ye Z, Xu Q, Li J, Liu N, Du Y. Luo Y, et al. Front Surg. 2022 Oct 14;9:988843. doi: 10.3389/fsurg.2022.988843. eCollection 2022. Front Surg. 2022. PMID: 36311952 Free PMC article. Review.
-
Establishing a reproducible approach to study cellular functions of plant cells with 3D bioprinting.
Van den Broeck L, Schwartz MF, Krishnamoorthy S, Tahir MA, Spurney RJ, Madison I, Melvin C, Gobble M, Nguyen T, Peters R, Hunt A, Muhammad A, Li B, Stuiver M, Horn T, Sozzani R. Van den Broeck L, et al. Sci Adv. 2022 Oct 14;8(41):eabp9906.
doi: 10.1126/sciadv.abp9906. Epub 2022 Oct 14. Sci Adv. 2022. PMID: 36240264 Free PMC article.
-
3D-printed microrobots from design to translation.
Dabbagh SR, Sarabi MR, Birtek MT, Seyfi S, Sitti M, Tasoglu S. Dabbagh SR, et al. Nat Commun. 2022 Oct 5;13(1):5875. doi: 10.1038/s41467-022-33409-3. Nat Commun. 2022. PMID: 36198675 Free PMC article. Review.
-
Skeletal Muscle Pathogenesis in Polyglutamine Diseases.
Marchioretti C, Zuccaro E, Pandey UB, Rosati J, Basso M, Pennuto M. Marchioretti C, et al. Cells. 2022 Jul 3;11(13):2105. doi: 10.3390/cells11132105. Cells. 2022. PMID: 35805189 Free PMC article. Review.
See all "Cited by" articles
References
-
- Exp Cell Res.
2010 Apr 15;316(7):1159-68 - PubMed
- Exp Cell Res.
-
- Acta Biomater. 2016 Mar 1;32:170-177 - PubMed
-
- Annu Rev Biomed Eng. 2014 Jul 11;16:247-76 - PubMed
-
- Adv Healthc Mater. 2013 Mar;2(3):442-9 - PubMed
-
- J Am Coll Cardiol.
2010 Aug 3;56(6):510-20 - PubMed
- J Am Coll Cardiol.
Publication types
MeSH terms
Substances
3D Bioprinting of Living Tissues
Progress in drug testing and regenerative medicine could greatly benefit from laboratory-engineered human tissues built of a variety of cell types with precise 3D architecture. But production of greater than millimeter sized human tissues has been limited by a lack of methods for building tissues with embedded life-sustaining vascular networks.
Play
In this video, the Wyss Institute and Harvard SEAS team uses a customizable 3D bioprinting method to build a thick vascularized tissue structure comprising human stem cells, collective matrix, and blood vessel endothelial cells.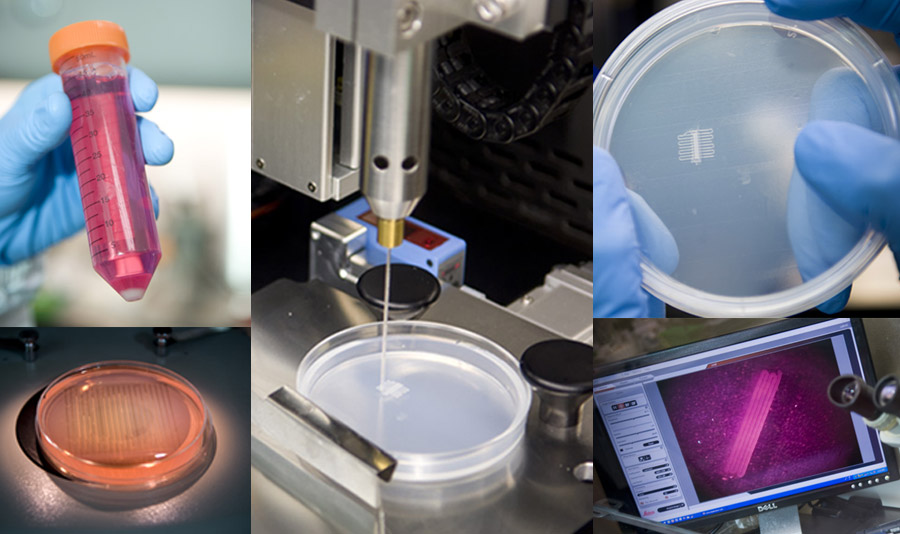
Multidisciplinary research at the Wyss Institute has led to the development of a multi-material 3D bioprinting method that generates vascularized tissues composed of living human cells that are nearly ten-fold thicker than previously engineered tissues and that can sustain their architecture and function for upwards of six weeks. The method uses a customizable, printed silicone mold to house and plumb the printed tissue on a chip. Inside this mold, a grid of larger vascular channels containing living endothelial cells in silicone ink is printed, into which a self-supporting ink containing living mesenchymal stem cells (MSCs) is layered in a separate print job. After printing, a liquid composed of fibroblasts and extracellular matrix is used to fill open regions within the construct, adding a connective tissue component that cross-links and further stabilizes the entire structure.
The resulting soft tissue structure can be immediately perfused with nutrients as well as growth and differentiation factors via a single inlet and outlet on opposite ends of the chip that connect to the vascular channel to ensure survival and maturation of the cells. In a proof-of-principle study, one centimeter thick bioprinted tissue constructs containing human bone marrow MSCs surrounded by connective tissue and supported by an artificial endothelium-lined vasculature, allowed the circulation of bone growth factors and, subsequently, the induction of bone development.
This innovative bioprinting approach can be modified to create various vascularized 3D tissues for regenerative medicine and drug testing endeavors. The Wyss team is also investigating the use of 3D bioprinting to fabricate new versions of the Institute’s organs on chips devices, which makes their manufacturing process more automated and enables development of increasingly complex microphysiological devices. This effort has resulted in the first entirely 3D-printed organ on a chip – a heart on a chip – with integrated soft strain sensors.
- 1/7 Cross section of long-term perfusion of HUVEC-lined (red) vascular network supporting HNDFladen (green) matrix.
- 2/7 Top-down view of long-term perfusion of HUVEC-lined (red) vascular network supporting HNDFladen (green) matrix.
- 3/7 Photograph cross section of printed tissue construct housed within a perfusion chamber.
- 4/7 Photograph cross section of printed tissue construct housed within a perfusion chamber.
- 5/7 Photograph of a printed tissue construct housed within a perfusion chamber.
- 6/7 Photograph of vasculature network and cell inks.
- 7/7 Photograph of 3D printed vasculature network (red) within Red is the
- Next
- Prev
Stem cells self-organized and became a material for 3D bioprinting
Scientists have developed a method for printing living tissues on a 3D bioprinter that uses stem cells and one of their most important properties is self-organization. As reported in Nature Materials , stem cells from various tissues placed in favorable conditions self-organized and formed tissues that looked and functioned like full-fledged living tissues.
The formation of tissues in a living organism depends on intercellular contacts and the microenvironment of cells. In the process of development and life activity, cells form around themselves an extracellular matrix - a part of the tissue that serves as a mechanical support and information intermediary for cells. Cells are located in the matrix (respectively, in the tissue) in a spatial relationship characteristic of each organ. To be in the right place at the right time, cells express hundreds of receptors and chemicals that determine how the cell interacts with neighboring cells and the matrix. Thanks to such interactions, cells self-organize - each cell knows where it needs to be in the tissue and what it needs to do.
Until recently, scientists were not able to grow large organelles (more than a centimeter) using 3D bioprinting, either because the cells were too tightly attached to the environment and could not move, or the environment itself did not allow creating the necessary microenvironment. However, Matthias P. Lutolf and colleagues at the Federal Polytechnic School of Lausanne have developed a new 3D bioprinting approach that can solve these problems. The new method, which, among other advantages, allows you to microscopically work with cell mass and directly observe the process of printing and growing, uses the self-organization of stem cells as the basis for growing full-fledged organs and tissues. This approach allows you to repeat the natural processes of development of tissues and organs.
To demonstrate the potential and versatility of the new method, the scientists used human small intestine stem cells. The line-printed stem cells were placed on a nutrient medium made of hydrogel with collagen, which is similar in properties to the extracellular matrix. In this environment, cells easily moved and created a fibrous connective tissue structure around themselves, additionally turning the environment into a favorable microenvironment.
A few days later, the cells transformed into a whole and organized epithelial tube 5 to 15 millimeters long, surrounded by a specific matrix, in which scientists found the tissue organization found in the classical organelles of the small intestine. At the same time, scientists note that it was the nutrient medium and the extracellular matrix that had a great influence on the formation of the intestinal tube, which created a favorable microenvironment for cell self-organization. It is noteworthy that intercellular self-organization leveled small printing defects (for example, cell adhesion).
The scientists also managed to grow the epithelium of the mouse small intestine. At first, the stem cells were arranged in a line, but after four or six days, thanks to the self-organization of the cells, a gap appeared in this line, which turned it into a hollow tube. After another one or two days, crypts and villi characteristic of the epithelium of the small intestine were found in the tube, in which scientists found mature differentiated enterocytes, Paneth cells (protective cells that are found only in the small intestine), goblet and enteroendocrine cells. The entire tube responded to external stimuli — Paneth cells released bactericidal granules in response to chemical stimulation, and all cells swelled under the action of forskolin . These reactions show that the new bioprinting technique can produce engineered tissues with physiological responses reminiscent of those in living organisms.
In addition, endothelial cells printed on a mixture with vascular endothelial growth factor (VEGF) formed capillary vessels de novo . Due to favorable conditions (VEGF, loose medium), the formation of capillaries was triggered at the tissue scale, which led to the formation of a vasculature with a continuous lumen.
All of these experiments show that the specific local interactions that govern the self-organization of a small cell block can extend to the tissue level and form different types of tissues: both endothelial and tissues of the internal environment. Using the property of self-organization of stem cells should be an important step towards growing tissues and organs in vitro , because in this case it will be possible to obtain functionally complete organs that can be used for transplantation or drug testing.
Recently, we told that another group of scientists from the same Swiss school has developed a 3D bioprinting method that differs from traditional layer-by-layer 3D printing in that the organ model is created simultaneously over the entire volume in one step, which reduces printing time to several tens of seconds.
Vyacheslav Gomenyuk
Found a typo? Select the fragment and press Ctrl+Enter.
3D printing of heart muscle cells / Sudo Null IT News
Heart cells under a microscope.
The dream of 3D printing heart tissue is one step closer, thanks to the development of scientists from the Heart Research Institute (HRI), Sydney, Australia.
Article by Sophie Scott from abc.net.au translated for you by Top 3D Shop .
Key points:
- Scientists hope artificial tissues can replace those damaged by heart attacks;
- Cells behave like real ones, they beat and move;
- Researchers hope the technology will be available to patients within the next five years.
Scientists are using a new bioprinter to print cells they say could replace a patient's damaged heart cells.
“The process will go something like this: when a patient enters the clinic, a tissue sample is taken from him, namely the skin, from which we extract cells. Based on them, stem cells are first generated, and from them - heart cells,"
says Dr. Carmine Gentile.
Living stem cells are printed on a base that will be "glued" directly onto the areas of the patient's heart damaged during an attack.
Heart Research Institute's cells contract together - "beat" like a real heart.
Pictured: heart cells grown from a tissue sample from a guinea pig.
“They act like a real heart. We were able to make this amazing discovery in our laboratory,"
said Dr. Gentile.
The success of the project could radically change how doctors treat people with heart attacks. Now patients after a heart attack are treated with angioplasty - to expand blocked or narrowed coronary arteries, a metal mesh balloon is inserted into them, which prevents the artery from sticking together and allows blood to circulate. Doctors also use reperfusion therapy - they prescribe drugs that destroy clots that block arteries. But this treatment is not suitable for all patients, says cardiologist Gemma Figtree of the Colling Institute.
3D printer of the Heart Research Institute.
“We don't know how to replace a healed muscle or what to use for heart regeneration. Currently, this is only one of the methods for studying the cardiovascular system, and this is only the first potential solution ",
- she said.
A bioprinter developed in Australia could be a lifesaver for these patients. Associate Professor Figtry believes that eventually the heart can be repaired.
“By replacing dead heart muscle with an effective patch, we can reduce heart failure, which will reduce shortness of breath and improve the quality of life for patients.”
According to statistics, there are 350,000 heart attack survivors in Australia.
Despite improvements in cardiovascular disease prevention, heart attacks kill 24 people every day in Australia.