3D body part printing
3D printing of body parts is coming fast – but regulations are not ready
In the last few years, the use of 3D printing has exploded in medicine. Engineers and medical professionals now routinely 3D print prosthetic hands and surgical tools. But 3D printing has only just begun to transform the field.
Today, a quickly emerging set of technologies known as bioprinting is poised to push the boundaries further. Bioprinting uses 3D printers and techniques to fabricate the three-dimensional structures of biological materials, from cells to biochemicals, through precise layer-by-layer positioning. The ultimate goal is to replicate functioning tissue and material, such as organs, which can then be transplanted into human beings.
We have been mapping the adoption of 3D printing technologies in the field of health care, and particularly bioprinting, in a collaboration between the law schools of Bournemouth University in the United Kingdom and Saint Louis University in the United States. While the future looks promising from a technical and scientific perspective, it’s far from clear how bioprinting and its products will be regulated. Such uncertainty can be problematic for manufacturers and patients alike, and could prevent bioprinting from living up to its promise.
From 3D printing to bioprinting
Bioprinting has its origins in 3D printing. Generally, 3D printing refers to all technologies that use a process of joining materials, usually layer upon layer, to make objects from data described in a digital 3D model. Though the technology initially had limited applications, it is now a widely recognized manufacturing system that is used across a broad range of industrial sectors. Companies are now 3D printing car parts, education tools like frog dissection kits and even 3D-printed houses. Both the United States Air Force and British Airways are developing ways of 3D printing airplane parts.
The NIH in the U.S. has a program to develop bioprinted tissue that’s similar to human tissue to speed up drug screening.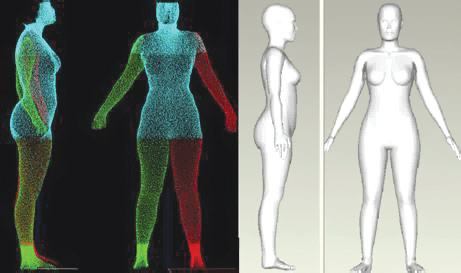
In medicine, doctors and researchers use 3D printing for several purposes. It can be used to generate accurate replicas of a patient’s body part. In reconstructive and plastic surgeries, implants can be specifically customized for patients using “biomodels” made possible by special software tools. Human heart valves, for instance, are now being 3D printed through several different processes although none have been transplanted into people yet. And there have been significant advances in 3D print methods in areas like dentistry over the past few years.
Bioprinting’s rapid emergence is built on recent advances in 3D printing techniques to engineer different types of products involving biological components, including human tissue and, more recently, vaccines.
While bioprinting is not entirely a new field because it is derived from general 3D printing principles, it is a novel concept for legal and regulatory purposes. And that is where the field could get tripped up if regulators cannot decide how to approach it.
State of the art in bioprinting
Scientists are still far from accomplishing 3D-printed organs because it’s incredibly difficult to connect printed structures to the vascular systems that carry life-sustaining blood and lymph throughout our bodies. But they have been successful in printing nonvascularized tissue like certain types of cartilage. They have also been able to produce ceramic and metal scaffolds that support bone tissue by using different types of bioprintable materials, such as gels and certain nanomaterials. A number of promising animal studies, some involving cardiac tissue, blood vessels and skin, suggest that the field is getting closer to its ultimate goal of transplantable organs.
Researchers explain ongoing work to make 3d-printed tissue that could one day be transplanted into a human body.We expect that advancements in bioprinting will increase at a steady pace, even with current technological limitations, potentially improving the lives of many patients. In 2019 alone, several research teams reported a number of breakthroughs. Bioengineers at Rice and Washington Universities, for example, used hydrogels to successfully print the first series of complex vascular networks. Scientists at Tel Aviv University managed to produce the first 3D-printed heart. It included “cells, blood vessels, ventricles and chambers” and used cells and biological materials from a human patient. In the United Kingdom, a team from Swansea University developed a bioprinting process to create an artificial bone matrix, using durable, regenerative biomaterial.
‘Cloneprinting’
Though the future looks promising from a technical and scientific perspective, current regulations around bioprinting pose some hurdles. From a conceptual point of view, it is hard to determine what bioprinting effectively is.
Consider the case of a 3D-printed heart: Is it best described as an organ or a product? Or should regulators look at it more like a medical device?
Regulators have a number of questions to answer. To begin with, they need to decide whether bioprinting should be regulated under new or existing frameworks, and if the latter, which ones. For instance, should they apply regulations for biologics, a class of complex pharmaceuticals that includes treatments for cancer and rheumatoid arthritis, because biologic materials are involved, as is the case with 3D-printed vaccines? Or should there be a regulatory framework for medical devices better suited to the task of customizing 3D-printed products like splints for newborns suffering from life-threatening medical conditions?
In Europe and the U.S., scholars and commentators have questioned whether bioprinted materials should enjoy patent protection because of the moral issues they raise. An analogy can be drawn from the famed Dolly the sheep over 20 years ago. In this case, it was held by the U.S. Court of Appeals for the Federal Circuit that cloned sheep cannot be patented because they were identical copies of naturally occurring sheep. This is a clear example of the parallels that exist between cloning and bioprinting. Some people speculate in the future there will be ‘cloneprinting,’ which has the potential for reviving extinct species or solving the organ transplant shortage.
Dolly the sheep’s example illustrates the court’s reluctance to traverse this path. Therefore, if, at some point in the future, bioprinters or indeed cloneprinters can be used to replicate not simply organs but also human beings using cloning technologies, a patent application of this nature could potentially fail, based on the current law. A study funded by the European Commission, led by Bournemouth University and due for completion in early 2020 aims to provide legal guidance on the various intellectual property and regulatory issues surrounding such issues, among others.
On the other hand, if European regulators classify the product of bioprinting as a medical device, there will be at least some degree of legal clarity, as a regulatory regime for medical devices has long been in place. In the United States, the FDA has issued guidance on 3D-printed medical devices, but not on the specifics of bioprinting. More important, such guidance is not binding and only represents the thinking of a particular agency at a point in time.
Cloudy regulatory outlook
Those are not the only uncertainties that are racking the field. Consider the recent progress surrounding 3D-printed organs, particularly the example of a 3D-printed heart. If a functioning 3D-printed heart becomes available, which body of law should apply beyond the realm of FDA regulations? In the United States, should the National Organ Transplant Act, which was written with human organs in mind, apply? Or do we need to amend the law, or even create a separate set of rules for 3D-printed organs?
We have no doubt that 3D printing in general, and bioprinting specifically, will advance rapidly in the coming years. Policymakers should be paying closer attention to the field to ensure that its progress does not outstrip their capacity to safely and effectively regulate it. If they succeed, it could usher in a new era in medicine that could improve the lives of countless patients.
[ You’re smart and curious about the world. So are The Conversation’s authors and editors. You can get our highlights each weekend. ]
3D Printers Can Already Create Human Body Parts
Do you feel like you are living in a sci-fi version of the future? Because you probably should.
A close up view of the skin bioprinter nozzle.WFIRM
In recent years, updates in 3D printing technologies have allowed medical researchers to print things that were not possible to make using the previous version of this technology, including food, medicine, and even body parts.
In 2018, doctors from the Ontario Veterinary College 3D printed a custom titanium plate for a dog that had lost part of its skull after cancer surgery.
Source: University of Guelph“By performing these procedures in our animal patients, we can provide valuable information that can be used to show the value and safety of these implants for humans”, said veterinary surgical oncologist Michelle Oblak at the time. “These implants are the next big leap in personalized medicine that allows for every element of an individual’s medical care to be specifically tailored to their particular needs.”
And not just for animal patients.
What is 3D bioprinting?
3D bioprinting is the utilization of 3D printing technologies to fabricate body parts. Bioprinters work in a similar way to 3D printers. However, instead of depositing materials such as plastic or ceramic, they deposit layers of biomaterial, including living cells, to build complex structures like blood vessels or skin tissue.
The required cells are taken from a patient and then cultivated. These cells are usually combined with a carrier material or scaffold. This carrier is usually a type of biopolymer gel, which acts as a 3D molecular scaffold and provides protection for the cells during the printing process. Cells attach to the gel, which is sturdy enough to allow printing and flexible enough to allow the flow and diffusion of nutrients and the movement of cells. This combination of encapsulated cells and biopolymer gels is the bio-ink used by biomedical engineers to create 3D-printed, tissue-like structures.
Detailed computer designs and models are first made, often based on scans such as magnetic resonance imaging or computerized tomography taken directly from a patient. Precision printer heads then deposit cells and bio-inks exactly where they are needed and, over the course of several hours, an organic object is built up using a large number of very thin layers.
A research fellow, Dr. Young Joon Seol, works on a project to print experimental muscle tissue for reconstructive surgery. Source: Army Medicine/FlickrThe cells are kept alive using liquefied nutrients and oxygen during the whole process.
In the post-printing stage, the structures may be crosslinked with UV light or ionic solutions to make them more stable. Cells are chemically and mechanically stimulated to control the remodeling and growth of tissues. Then, the 3D printed product is put into an incubator to allow the cells to grow.
When it’s ready, the structure must be used as soon as possible, unless the 3D bioprinting is combined with cryopreservative techniques — something that researchers from Brigham and Women's Hospital and Harvard Medical School achieved last year.
Most Popular
The work, published on December 21st, showed how the team was able to 3D print tissues onto a cold plate at -4°F (-20ºC), after which they were preserved in a freezer at -320.8°F (-196ºC). The tissues, researchers said, can then be thawed within minutes for immediate use.
Tendons and ligaments
In 2018, biomedical engineers from the University of Utah developed a method for 3D printing ligaments and tendons. The method involves first taking stem cells from the patient and printing them on a layer of hydrogel to form a tendon or ligament. This is allowed to grow in vitro in a culture before being implanted. However, the process was very complex, because connective tissue is made up of different cells in complex patterns. The team first needed to develop a special printer head that could lay down human cells in the highly controlled manner they require.
To do this, the team partnered with Utah-based company Carterra, Inc., to develop a specialized printhead that would let them lay down cells in complex patterns. The printhead was then attached to a 3D printer normally used to print antibodies for cancer treatment.
With this technique, the scientists managed to 3D print stem cells taken from a patient’s body fat onto a layer of hydrogel. This hydrogel facilitates cell growth in vitro in a culture, forming either a ligament or tendon in the process.
The new tissue is then implanted in the damaged area of the patient’s body, eliminating the need for additional tissue replacement procedures.
Replacement tissues for those needing it are often harvested from elsewhere on a patient's body or from a cadaver. However, tissue from cadavers runs a high risk of being rejected by the surrounding tissues or of being of poor quality and ineffective.
Instead, tissues created from the patient’s own cells can reduce the complications involved with a transplant and speed up the healing process.
Skin bioprinting and wound healing
3D bioprinting could also help us say goodbye to skin grafts in the near future, as doctors could be able to 3D print new skin for each patient.
Skin grafting is the transplantation of healthy skin from an animal, a human donor, or the patient’s own body to another part of his or her body where the skin is badly damaged. The procedure is commonly used to treat severe wounds, burns, ulcers, and infections, or after the removal of skin cancers.
Source: Scientific Animations/Wikimedia CommonsBut the technique involves several risks, from hemorrhages and loss of sensitivity to infections, scarring, and rejection.
This is why scientists from Wake Forest Institute for Regenerative Medicine (WFIRM) are working on a mobile bedside skin bioprinting system that could let doctors print bi-layered skin directly on the patient’s wound.
“The unique aspect of this technology is the mobility of the system and the ability to provide on-site management of extensive wounds by scanning and measuring them in order to deposit the cells directly where they are needed to create skin,” said Sean Murphy, Ph.D., a WFIRM assistant professor who was the lead author of the paper.
A WFIRM technician operates the mobile bioprinter for skin printing on a limb demo. Source: WFIRMTo do this, the scientists isolated certain skin cells from a biopsy of healthy tissue and grew them in culture. After that, they combined the cells with a hydrogel and put them into the bioprinter. The device printed the cells onto the damaged area following the data extracted from the wound’s scan through a software.
Again, because the cells are taken from the patient’s own body, there is a much lower risk of rejection.
Meanwhile, in Dublin, scientists from the RCSI University of Medicine and Health Sciences developed a hydrogel scaffold with natural platelet-rich plasma (PRP) that has promising regenerative properties. The compound can be used as a bio-ink to accelerate the wound healing process in 3D printed tissues.
“Existing literature suggests that while the PRP already present in our blood helps to heal wounds, scarring can still occur,” said RCSI Professor Fergal O’Brien. “By 3D printing PRP into a biomaterial scaffold, we can increase the formation of blood vessels while also avoiding the formation of scars, leading to more successful wound healing.”
Blood vessels
Perhaps the ultimate goal of 3D bioprinting is to assemble functional organs and solve the problem of organ transplantation.
Currently, there are more than 100,000 people waiting for an organ on the U.S. national transplant waiting list. Roughly 17 of them die each day because they don’t receive the organ they need. This is largely due to the lack of donors. Although around 60% of Americans are signed up as donors, organ donation is only possible in 3 out of every 1,000 deaths.
3D bioprinting of organs could save a lot of lives, but scientists struggle to create the vascular structures needed to create viable printed organs. All organs, including 3D-printed ones, need an effective, continuous blood supply to prevent the death of the cells and the tissues.
In October 2021, a team of researchers at Israel's Technion Institute of Technology managed to 3D print blood vessel structures to add a blood supply to tissue implants.
These structures grew spontaneously after the team implanted endothelial cells from the inner layer of blood vessels in the body in a polymeric collagen scaffold.
However, these are only microvessels that can be used to improve in vitro tissue development — they wouldn’t be able to, “feed” a whole organ, and so far, they don’t allow the integration of lab-grown tissues into the patient’s vascular system.
This study shows that there’s still a long way to go until we can actually 3D print organs on demand. But who knows which techniques can scientists develop to solve this issue in the future?
More Stories
innovation
The 'world’s largest' solar power+storage project will displace 1. 4M tons of coal
Derya Özdemir| 6/9/2022
diy
Learn how to make a basic wooden robotic grabber arm with this guide
Christopher McFadden| 3/20/2022
culture
Here’s why the Turkish government wants to build another Bosphorus
Eric James Beyer| 3/10/2022
Organ printing: how 3D bioprinting technology has advanced and what is hindering its development In research centers and hospitals around the world, advances in 3D printing and bioprinting are providing new opportunities for human treatment and scientific research. In the coming decades, bioprinting could be the next major milestone in healthcare and personalized medicine.
Let's talk about bioprinting technology, the latest advances in the industry and the limitations that professionals face.
How a 3D printer works
Traditional printers, like the one you have at home or office, work in two dimensions. They can print text or images on a flat surface (usually paper) using the x (horizontal) and y (vertical) dimensions. 3D printers add another dimension - depth (z). During the printing process, the printer heads can move up and down, left and right, back and forth, but instead of delivering ink to paper, they distribute various materials - polymers, metal, ceramics and even chocolate - until the "print" of a holistic, voluminous object , layer by layer in a process known as "additive manufacturing".
To create a 3D object, you need a blueprint for it, a digital file created with modeling software. After its creation, the computer-generated model is sent to the printer. Your chosen material is loaded into the machine and ready to be heated to easily flow out of the printer nozzle. As the printer reads the plan, its head moves, depositing successive layers of the selected material to create the final product.
As each layer is printed, it is solidified either by cooling or by mixing two different solutions delivered by the printer head. The new layers precisely lay down on the previous ones to make a stable, cohesive element. In this way, you can create almost any shape, including a moving one.
3D printing allows you to create objects with geometric structures that would be difficult or impossible to make in other ways. A wide range of products are already being created using 3D printers, including jewelry, clothing, toys, and high-end industrial products. Even a 10-year-old Moscow schoolboy has learned how to work with a 3D printer: he prints 3D figures to order and sells them through Instagram.
How a bioprinter works
Bioprinters work in much the same way as 3D printers, with one key difference - they deposit layers of biomaterial, which can include living cells, to create complex structures such as blood vessels or skin tissue.
Living cells? Where do they get them? Every tissue in the body is made up of different types of cells. The required cells (kidney, skin, and so on) are taken from the patient and then cultured until there are enough of them to create "bio-ink" that is loaded into the printer. This is not always possible, therefore, for some tissues, stem cells are taken that are capable of becoming any cell in the body (organism), or, for example, porcine collagen protein, seaweed and others.
Often used in bioprinting is chitosan, a polysaccharide obtained from the external skeleton of mollusks (eg shrimp) or by fermenting fungi. This material has high biocompatibility and antibacterial properties. Its disadvantage is the low rate of gelation. Another popular material is a polysaccharide isolated from seaweed called agarose. Its advantages are high stability and the possibility of non-toxic cross-linking during research. However, this biomaterial does not decompose and has poor cell adhesion (the ability of cells to stick together with each other and with other substrates).
Collagen, a primary structural protein found in the skin and other connective tissues, has a high biological significance. It is the most abundant protein in mammals and a major component of connective tissue. Its disadvantages for bioprinting include the property of acid solubility. More information about biomaterials can be found here.
Based on computer designs and models, often scans and MRIs taken directly from the patient, the printer heads place the cells exactly where they are needed and within a few hours an organic object is built from a large number of very thin layers.
Organovo bioprinter creates tissues that mimic the structure and composition of various human organs
Source: Pbs.org
Scaffolding for ear or nose replacement at Wake Forest University in Winston-Salem, North Carolina
Source: CBS News
Computer displays an image of a "scaffold" for the human ear, created in the laboratory of Wake Forest University in Winston-Salem, North Carolina
Source: CBS News
Usually more than just cells are needed, so most bioprinters also supply some kind of organic or synthetic "glue" - a soluble gel or collagen scaffold to which cells can attach and grow. This helps them form and stabilize in the correct shape. Surprisingly, some cells can take the correct position on their own without any "scaffolding". How do they know where to go? How do embryonic cells develop in the uterus, or does adult tissue move to repair damage? Same here.
Universities, researchers and private companies around the world are involved in the development of bioprinting technologies. Let's take a look at some of the amazing things they are working on.
Bioprinting in Russia
3D Bioprinting Solutions is a biotechnology research laboratory founded by medical company INVITRO. The activity of the laboratory is the development and production of bioprinters and materials in the field of three-dimensional bioprinting and scientific research. August 23, 20193D Bioprinting Solutions laboratory sent a new batch of cuvettes to the ISS to continue experiments on bioprinting in space, which began in 2018. This was reported in the press center of the laboratory. This time it is planned to use organic and inorganic components to assemble bone tissue on the world's first space bioprinter Organ.Aut.
Symposium "Biofabrication in Space"
Source: Zdrav.Expert
Organ.Aut magnetic bioprinter
Source: Zdrav.Expert
The astronauts will also grow protein crystals and experiment with printing biofilms of bacteria to study their behavior in zero gravity. Russian scientists expect to receive unique scientific data that can be applied in the development of new drugs.
Scientific director of 3D Bioprinting Solutions and leading researcher of the Institute of Regenerative Medicine, Candidate of Medical Sciences Vladimir Mironov, in his speech at the Department of Anatomy of Sechenov University on September 2, noted: “Living cells, tissues and human organs will be synthesized already in the current century. To do this, morphological sciences, such as microscopic anatomy and histology, must be digitized or digitalized, that is, digitized and made available for computer programs of robotic bioprinters, since without digital models it is impossible to print human tissues and organs. ”
Bioprinting around the world
Every year, millions of people around the world need bone grafting. Modern bone grafts often use cement-based synthetic material in combination with the patient's own bone. However, the use of these materials has a number of limitations - some transplants caused rejection and inflammatory processes in patients. Reproduction of the natural bone-cartilage "interface" has also been problematic.
However, a team at Swansea University in 2014 developed a bioprinting technology that allows the creation of an artificial bone prosthesis in the exact shape of the desired bone, using a biocompatible material that is both durable and regenerative. At the same time, scientists from the University of Nottingham in England were working on similar studies.
It takes about two hours to print a small bone. Therefore, surgeons can do it right in the operating room. This part of the bone is then covered with adult stem cells that can develop into almost any other type of cell. This is combined with bio-ink from the printer, a combination of polylactic acid (which provides mechanical strength to bone) and alginate, a gel-like substance that serves as a shock-absorbing material for cells. The end product is then implanted into the body, where it will completely disappear within about three months and be replaced by new bone.
Researchers hope that in the future, bioprinted bones can be created with sufficient reliability to support complex spinal reconstruction, and that the bone material will be further improved to increase its compatibility with cartilage cells.
Source: ETH Zurich
Successful 3D printing of human cartilage may soon completely replace artificial implants for people in need of reconstructive surgery. Back in 2015, scientists in Zurich developed technology that would allow hospitals to print a full-size human nose implant in less than 20 minutes. They believe that any cartilage implant can be made using their technique.
Researcher Matti Kesti described the technology as follows:
“
“A serious car accident can cause the driver or passenger to suffer complex nose injuries. The nose can be restored by creating a 3D model on a computer. At the same time, a biopsy of the patient is performed and cartilage cells are removed from the victim's body, such as from a knee, a finger, an ear, or fragments of a broken nose. The cells are spawned in the laboratory and mixed with the biopolymer. From this suspension, a model of nasal cartilage is created using a bioprinter, which is implanted into the patient during surgery. In the process, the biopolymer is used simply as a mold. It is subsequently broken down by the body's own cartilage cells. And in a couple of months it will be impossible to distinguish between the graft and the person’s own nasal cartilage.”
Matti Kesti
Since the implant was grown from the body's own cells, the risk of rejection will be much lower than for an implant made of, say, silicone. An additional advantage is that the bioimplant grows with the patient, which is especially important for children and young adults.
If a person is severely burned, healthy skin can be taken from another part of the body and used to cover the affected area. Sometimes intact skin is missing.
Researchers at Wake Forest School of Medicine have successfully designed, built and tested a printer that can print skin cells directly onto a burn wound. The scanner very accurately determines the size and depth of damage. This information is sent to a printer and skin is printed to cover the wound. Unlike traditional skin grafts, it only takes a patch of skin one-tenth the size of a burn to grow enough cells to print. While this technology is still in the experimental stage, the researchers hope that it will be widely available within the next five years.
As already mentioned, 3D printers print products in layers, and since the skin is a multi-layered organ with different types of cells, it is well suited for this type of technology. However, researchers still have a lot of problems to solve, in particular, how to prevent damage to cells from the heat generated by the printer. And of course, like most parts of the human body, the skin is more complex than it first appears—there are nerve endings, blood vessels, and a host of other aspects to consider.
Blood vessels
Biomechanical engineer Monica Moya holding a petri dish with printed alginate-based biotubes. Biotubes can act as temporary blood vessels similar to blood vessels that help create a patch of living tissue.
Source: embodi3D
With tens of thousands of miles of veins, arteries and capillaries in the human body, researchers are working to replace them if they ever wear out. The creation of viable blood vessels is also essential for the proper functioning of all other potential bioprinted body parts.
Biomechanical Engineer Monica Moya of Livermore National Laboratory. Lawrence uses bioprinting to create blood vessels. The materials created by her bioprinters are engineered to allow small blood vessels to develop on their own.
This development takes time, so vials of cells and other biomaterials are printed to help deliver vital nutrients to the printed environment. After a while, self-assembled capillaries connect with bioprinted tubes and begin to deliver nutrients to cells on their own, mimicking the work of these structures in the human body.
Internal organs
Many researchers hope that in 20 years the lists of patients waiting for organ transplants will become a thing of the past. They envision a world where any organ can be printed and transplanted in just a few hours, without rejection or complications, because these organs will be created from body cells according to the individual characteristics of each patient. Currently, bioprinting of fully functional complex internal organs is not possible, but research is ongoing (and not without success).
Bladder
For example, the bladder is already printed. In 2013, at Wake Forest University in the US, researchers successfully took cells from a patient's original, poorly functioning bladder, cultured them, and added additional nutrients. The 3D shape of the patient's bladder was then printed and the cultured cells soaked through it. The form was placed in an incubator and, when it reached the desired condition, it was transplanted into the patient's body. The mold will eventually collapse, leaving only the organic material. The same team successfully created viable urethras.
Physicians and scientists at the Wake Forest Institute for Regenerative Medicine (WFIRM) were the first in the world to create laboratory-grown organs and tissues that were successfully transplanted into humans. Right now they are working on growing tissues and organs for more than 30 different areas of the body, from the kidneys and trachea to cartilage and lungs. They also aim to accelerate the availability of these treatments to patients.
Scientists in Australia are doing similar research as well. They used human stem cells to grow a kidney organ that contains all the necessary cell types for a kidney. Such cells can serve as a valuable initial source for bioprinting more complex kidney structures.
MD, Professor of Urology, Professor of the Institute of Regenerative Medicine Anthony Atala shows a kidney created by a bioprinter. A modified desktop inkjet printer sprays cells instead of ink. The cells were cultured from the patient and the structural template for the kidney was obtained from the MRI (so it is the correct size and shape).
Using this technology, back in 2001, Atala printed and successfully transplanted a bladder into a young man, Jake.
Source: TedEd
Heart
Heart cells, laboratory-grown organelles. Source
Surprisingly, it is the human heart that can become one of the easiest organs to print, since, in fact, it is a pump with tubes. Of course, everything is not so simple, but many researchers believe that humanity will learn to print hearts before kidneys or liver.
Researchers at the Wake Forest Institute for Regenerative Medicine in April 2015 created "organoids" - 3D printed fully functional, beating heart cells.
In April 2019, Israeli scientists printed the world's first 3D heart. It is still very small, the size of a cherry, but it is able to perform its functions. The 3D heart with blood vessels uses personalized "ink" of collagen, a protein that supports cell structures, and other biological molecules.
A Tel Aviv University researcher holds the world's first 3D printed heart on April 15, 2019.
Source: Haaretz
“This is the first time anyone anywhere has successfully designed and printed a whole heart with cells, blood vessels, ventricles and chambers,” said Tel Aviv University scientist Professor Tal Dvir.
So far, scientists have been able to print tissue from cartilage and the aortic valve, for example, but the challenge has been to create tissue with vascularity—the blood vessels, including capillaries, without which organs cannot survive, let alone function.
The Tel Aviv scientists started with human adipose tissue and separated the cellular and non-cellular components. They then reprogrammed the cells to become undifferentiated stem cells, which could then become cardiac or endothelial. Endothelium - a single layer of flat cells lining the inner surface of the heart cavities, blood and lymphatic vessels. Endothelial cells perform many functions of the vascular system, such as controlling blood pressure, regulating the components of blood clotting, and the formation of new blood vessels.
Non-cellular materials, including a large amount of proteins, were processed into a "personalized hydrogel" that served as "printing ink".
It will be years before this technology can create organs for efficient transplantation. However, the achievements of scientists in Tel Aviv are a huge milestone along the way.
Medical research and pharmacology
One of the key potential uses for bioprinted living materials is in the field of medical and drug research. Bioprinted tissues have several cell types with different densities and key architectural features. This allows researchers to study the impact of various diseases on the body, the stages of disease progression and possible treatments in the natural microenvironment.
One of the most impressive developments in recent years is the development of a desktop brain at the ARC Center of Excellence in 2016. The researchers were able to use a 3D printer to create a 3D printed six-layer structure that includes nerve cells that mimic the structure of brain tissue.
This opens up huge potential benefits for researchers, pharmaceuticals and private companies, because it will allow them to test new products and drugs on tissue that accurately reflects the responses of human brain tissue, as opposed to animal samples, which may cause a completely different response. The desktop brain can also be used to further investigate diseases such as schizophrenia or Alzheimer's.
We are far from printing the brain, but the ability to arrange cells to form neural networks is a significant step forward. By allowing researchers to work with human tissue in real time, testing processes can be greatly accelerated and results can be more realistic and accurate. It will also reduce the need to use laboratory animals for medical tests and potentially dangerous human testing.
Medical simulators and data registries
Source: Simbionix
About 3,000 medical simulators are currently in use around the world to help doctors practice complex procedures. Virtual blood vessels, 3D printed organs... and no animal suffers!
The American company 3D Systems created an industry segment called VSP (Virtual Surgical Planning). This approach to personalized surgery combines expertise in medical imaging, surgical simulation and 3D printing. Surgeons using the Simbionix medical simulator for the first time often report feeling physical pain while empathizing with their virtual patient - the experience is so realistic. Organs and tissues look completely real. When stitching an organ, the surgeon sees on the screen a needle that enters the tissue, and pulls the thread. If the doctor does something wrong, the virtual blood vessels break and the organ begins to bleed. These simulators were developed by the Israeli company Symbionix, which was acquired by 3D Systems in 2014.
On September 3, 2019, the Radiology Society of North America (RSNA) and the American College of Radiology (ACR) announced the launch of a new 3D Medical Printing Clinical Data Registry to collect data on treatment outcomes using 3D printing at the point of care. This information will be a powerful tool to assess and improve patient care in real time, drive ongoing research and development, and inform patients and healthcare professionals about the best course of care.
“
“The creation of a joint RSNA-ACR 3D printing registry is essential to the advancement of clinical 3D printing. The registry will collect data to support the appropriate use of this technology and its implications for clinical decision making. ”
William Widock, Professor of Radiology at the University of Michigan and Chairman of the RSNA 3D Printing Special Interest Group (SIG)
According to RSNA, the information in the registry will allow for the necessary analysis to demonstrate the clinical value of 3D printing. Due to the wide variety of clinical indications, different technologies for creating physical models from medical images, and the complexity of the models, it is problematic to choose the optimal treatment method. The registry will help solve this problem.
Bioprinting software
Bioprinter and bioprinting software manufacturer Allevi introduced Allevi Bioprint Pro software on September 5, 2019. Built-in model generation and integrated slicing will allow you to focus more on experimenting, rather than setting up the printer. The program runs entirely in the cloud, which means you can create your biostructures, define materials, and track prints right from a web browser on any computer.
According to the development team, the new bioprinter with the above software is powerful and easy to use and represents another piece of the puzzle on the way to 3D printed organs.
At the same time, CELLINK, the first bio-ink company, announced the launch of a new product to become the most flexible bio-printing platform on the market. The BIO X6 bioprinter, which has no analogues at the moment, has the ability to combine more bioprinting materials, cells and tools.
Why is this taking so long?
Complex body structure
The human body and its various components are much more complex than a plastic toy. The human organ has a complex network of cells, tissues, nerves, and structures that must be arranged in specific ways to function properly. From placing thousands of tiny capillaries in the liver to actually getting a printed heart that "beats" and contracts in the human body, there is still a lot of research and testing.
Legal regulation
In addition, bioprinting technologies, like all new medical treatments, must pass safety tests and due process of regulation before they become available.
Special software and hardware
It also takes time to develop special software and hardware. These programs can be written only with the appropriate data (medical, clinical, statistical, mathematical, and so on), which someone must first collect, analyze, systematize and digitize.
Working through all of these steps requires the integration of technologies from various fields, including engineering, biomaterials science, cell biology, physics, mathematics, and medicine. So we need to be a little more patient.
The main thing is to know that those who work in the field, doctors and engineers, programmers and scientists are making progress every day both in bioprinting technology itself and in understanding how it can be used and improved. Although we are not quite there yet, there is no doubt that medicine will be very different in 10-20 years, thanks also to bioprinting.
In brief
Bioprinting is an extension of traditional 3D printing.
Bioprinting can produce living tissue, bones, blood vessels, and possibly entire organs for use in medical procedures, medical training, and testing.
The cellular complexity of a living organism has made 3D bioprinting slower to develop than conventional 3D printing.
Bioprinting technology could enable the generation of patient-specific tissues to develop precise, targeted and fully personalized treatments.
We still have a long way to go before we can create fully functioning and viable organs for human transplantation.
Related materials: Russia was the first in the world to print living tissues in space using a bioprinter
5 most amazing things created using 3D printing
A rocket printed on a 3D printerwill go into orbit in 2021 "Exhibits touching is allowed”: how 3D printing is transforming museums
© Rusbase, 2019
Author: Nadezhda Aleinik
Cover photo: etonastenka, Depositphotos
Body parts that can already be printed on a 3D printer – Blog Imena.
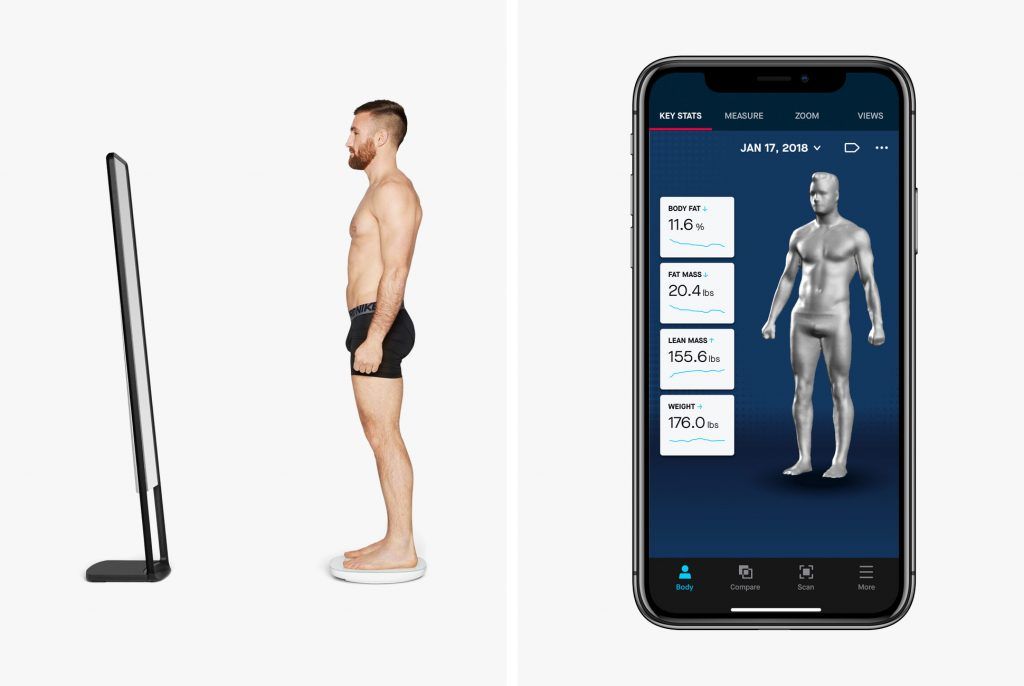
areas of human activity. Including in medicine. Presumably, with the help of this equipment, the problem of donation will be finally solved. After all, almost any part of the body or organ can simply be printed. And no matter how fantastic it may sound, scientists have already achieved amazing results in this direction.
Pinna
At least 1 in 12,500 newborns is born with microtia, a condition that can lead to hearing problems due to abnormal or missing pinna. In this case, surgical intervention is attempted, or a complete replacement of the shell may be required. Up to this point, artificial implants were used, which were often rejected by the body.
But using a 3D printer, it is already possible to create an auricle from living cells. The organ is first modeled in SolidWorks CAD software (and it is not difficult to create an exact copy of the other ear using 3D scanning), and then printed using a special high-density gel consisting of cells taken from bovine cartilage - they require about 25 million. Collagen (protein, providing strength and elasticity of the fabric) is “extracted” from rat tails. After the "printing" procedure, about 3 months of incubation period is required.
Being completely natural, this auricle is more readily accepted by the body. The chance of rejection is much lower. The technique was developed by scientists from Cornell University.
By the way, ears can be printed not only for the treatment of diseases or injuries. So, scientists from Princeton University are creating special ears that can pick up radio signals. It is assumed that such additions will be very useful for various electronics for the time being, and in the future they will be able to expand human capabilities.
Kidneys
As you know, with a sick heart or stomach, a person can live more or less normally for many years. Sick kidneys usually mean a significant reduction in life expectancy. However, it is sometimes extremely difficult or simply impossible to make a “replacement” due to the lack of a suitable donor. And the possibility of "conveyor" production of kidneys would save the lives of tens of thousands of people around the world.
Scientists from the Wake Forest Institute for Regenerative Medicine are currently working on 3D printing of kidneys. To create a new organ, cells are taken directly from the organs of the patient. That is, the chance that his body will more readily accept an implant that will not differ much from the replaced one increases significantly.
Unfortunately, this is only a project so far, but with very bright prospects. It is likely that in the very near future, scientists will learn how to create kidneys for "any requests."
Blood vessels
Teams of scientists from the University of Pennsylvania and the Massachusetts Institute of Technology are currently working on making printed blood vessels. For work, a printer developed by the RepRap project and software specially created for such purposes is used.
Future vessels are based on sugar, mold fungi and special polymers obtained from corn. Moreover, sugar is needed only for shaping, then it is washed out with water.
However, the obtained vessels cannot yet be used in the human body. But we are talking only about the development of the principles themselves. But the ability to create blood vessels that are indistinguishable from real ones is an important step towards creating any human organs using 3D printers.
Leather
Scientists from the Wake Forest Institute of Regenerative Medicine, already mentioned above, are also developing a technique when the skin will not be transplanted from other parts of the victim's body or from a donor, but printed directly on the affected area.
"Print" is preceded by a preliminary scan and mapping of the patient's wound. When creating the skin, various proteins and enzymes will be used, such as fibrinogen, collagen, thrombin, etc., each of which is then involved in the process of creating cells.
The ultimate goal is to create a compact 3D printer that can be used in disaster areas or in war.
Bones
Every year, thousands of people suffer complex bone fractures that are very difficult to repair using traditional methods. The technique, on which scientists from the University of Washington are currently working, is good because it will allow you to create replacements for any bones in the human body.
It was decided to use a special ceramic powder to create grafts. After printing is completed, the finished bone is subjected to heat treatment - heated to 2282 degrees for 120 minutes. The finished product is similar in its properties to human bone and can be used in the body.
In this case, the printing process is not too complicated. This technology is already actively used in the creation of some parts of electric motors. Thus, it is the printing of bones that looks like the most complete technology, the mass application of which is quite possible in the very near future.
By the way, this is not the first attempt to use 3D printers to print bone tissue.