How to cast metal parts from 3d prints
Introduction to Metal Casting and Ways to Combine 3D Printing With Casting Workflows
Metal casting is an age-old metalworking process in which molten metal cools and solidifies in a mold to form metal parts. Despite its ancient roots, metal casting is still one of the most popular processes for companies looking to produce metal parts.
This article will cover what metal casting is, how it works, and walks you through the most common metal casting processes and the benefits manufacturers can attain by combining modern digital tools like 3D printing with traditional casting workflows.
White Paper
Get design guidelines for creating 3D printed patterns, walk through the step-by-step direct investment casting process, and explore guidelines for indirect investment casting and sand casting.
Download the White Paper
Metal casting step-by-step from the original design through final casting.
Since the advent of metal casting, the methods have evolved and varied. Its core techniques, however, have remained constant. Here is a general step-by-step process for metal casting:
Ring patterns 3D printed in Castable Wax 40 Resin.
In order to begin the metal casting process, a manufacturer first must develop a representation of the desired pattern. This pattern is essential in designing the mold used for the cast. It is traditionally made from wood, foam, plastic, or wax and ensures that the mold accurately produces the finished metal part. Today, 3D printing is also a common method to produce patterns, which allows designers to create accurate patterns directly from digital CAD software tools.
A pattern is not an exact replica of the desired part. It has additional elements that make the casting process possible, including gates that allow molten metal to flow at a steady rate and vents for gas to escape. Additionally, patterns are also larger than the parts they represent to account for the shrinkage that occurs during cooling.
When the casting piece is hollow, the manufacturer also creates a core of sand or metal to shape the internal form. This core gets removed upon completion of the casting.
The next step is creating a casting mold, which can be either reusable (non-expendable) or non-reusable (expendable). Non-reusable molds are usually made out of sand, plaster, wax, or by 3D printing, and just as the name suggests, they get destroyed in the casting process. Reusable molds are made out of metal and other durable materials and can be reused for multiple casting cycles.
Ceramic shells after burnout and 3D printed patterns in Clear Resin.
Molten pewter poured into a High Temp Resin 3D printed mold for metal casting.
During this step, the metal gets heated in a furnace until it melts. Depending on the application, manufacturers can use a variety of different metals, with the most commonly cast metals being iron, aluminum, aluminum alloy, steel, copper, and zinc, as well as precious metals like gold and silver. Once the metal melts, the manufacturer pours it into the mold cavity and allows it to cool and solidify.
Metal casting post-production.
Once the metal cools down and solidifies, the parts get removed from the mold. Depending on the mold type, this can be done by vibrations in a shakeout process, washing away the investment material, or by ejector pins. Then, excess material, such as vents, gates, and feeders, are removed from the parts. Finally, the parts get filed, grated, machined, or sandblasted to smooth the surface and reach the final shape requirements.
Though all metal casting techniques share the same core process, there are various methods better suited for different applications. Some of the most common methods include die casting, investment casting, and sand casting.
Die casting uses a steel mold and high pressure. (Source: buhlergroup.com)
Die casting is a metal casting process in which a manufacturer pushes molten metal into a steel mold cavity at a high pressure to quickly produce metal parts. In die casting, the manufacturer fixes two halves of a die or reusable mold together and uses a nozzle to inject pressurized molten metal into the die. When the metal cools, the die opens, and ejector pins push out the cast.
The two most common die casting processes are hot-chamber and cold-chamber casting. While the specifics of these processes vary, there are several shared characteristics of the die casting process as a whole.
Hot-chamber die casting is the most common of the two main die casting processes. Hot-chamber die casting machines have a built-in furnace to heat the metal within the machine. Once the metal reaches a molten state, the machine lowers a cylindrical chamber into the molten metal. The gooseneck shape of the metal injection system allows the chamber to quickly fill itself, and then push the material into the mold with air pressure or a piston.
Immersing the injection mechanism to fill it allows for rapid and streamlined mold injection in this casting process. Because the chamber is subject to direct heat from the molten metal, however, hot-chamber die casting systems are at risk for corrosion, making them a less viable option for metals with high melting points. Instead, it is better suited for materials with low melting points and high fluidity, like lead, magnesium, zinc, and copper.
By contrast, the cold-chamber die casting process works more slowly to avoid corrosion. With this method, a foundry worker ladles molten metal into the injection system. A piston then pushes the metal into the mold.
This process limits the corrosion that is more common in hot-chamber die casting. It is an ideal option for metals with high melting points, like aluminum and aluminum alloy.
The die casting process is rapid and produces highly detailed parts. It is ideal for the production of high volumes of complex parts and can also produce strong parts with smooth surface finishes. Die casting’s capacity to produce a high volume of parts makes it a crucial process in the automotive and aerospace industries.
As die casting tooling and equipment are expensive, this process is not cost-effective for smaller production runs. In addition, the malleability of metals used in the process can impact the complexity of the product.
Cast parts from SLA patterns printed in Clear Resin on a Formlabs 3D printer.
Investment casting, also known as lost-wax casting, is a process that uses wax, slurry, and molds to produce complex parts. It is one of the oldest metal casting techniques but is still valued for its ability to create precise metal parts with intricate shapes.
This process is still widely used for producing jewelry, dentistry, and art. Its industrial form, investment casting, is a common way to create precision metal parts in engineering and manufacturing.
Investment casting patterns are typically made out of wax or 3D printed polymers. The patterns are assembled into a tree-like structure and dipped into a slurry of silica, or put into a flask and surrounded by the liquid investment plaster. After the investment material dries, the flask is placed upside down into a kiln, which melts the pattern, leaving a negative cavity in the shape of the original model. Metal is melted and then poured, using gravity or vacuum pressure to pull the metal into the cavity. The casted parts are filed, ground, machined, or sandblasted to achieve final geometry and surface finish.
Sprue trees with cast rings.
Investment casting is a versatile process. It allows manufacturers to produce accurate and repeatable parts out of nearly any metal available for casting and complicated shapes that would be difficult or impossible with other casting methods. Casted parts also have excellent surface qualities and low tolerances, with minimal surface finishing or machining required.
These features make investment casting ideal for complex parts in automotive, aerospace, and industrial applications, medical tools, dental implants, as well as fine jewelry and art.
Investment casting is a complex and labor-intensive process. It requires specialized equipment, costly refractories and binders, as well many manual operations to make a mold. It can be difficult to cast parts that require cores and the process is better suited to small parts.
One half of a sand casting mold.
Sand casting is a metal casting method that was first in use 3,000 years ago but remains the most widely used casting method to this day. This process allows manufacturers to cast metal without relying on machining.
In the sand casting process, the manufacturer first creates a foundry pattern, or replica of the casting, most commonly from wood or plastic. The pattern is oversized to allow for shrinkage. Parts with features on one side only require an open-faced mold. For parts with multiple detailed surfaces, the manufacturer separates the foundry pattern into two mold boxes to form a closed cavity mold. The top half is called a cope and the bottom a drag.
Once the manufacturer creates the pattern, they tightly pack sand around the pattern. Then, they add sprues and gates to ensure that the molten metal flows smoothly through the mold cavity. The manufacturer removes the pattern then clamps the two halves of the sand mold together. Once the metal melts to a molten state, it is poured into the mold and left to cool. From here, the sand mold is removed using vibrations or high-pressure water. Finally, the manufacturer refines the part by removing sprues and gates, and polishing the cast metal part.
Sand casting is an adaptable process that functions outside the limitations of machinery. Because of this, it can create complex parts of virtually any size. Sand is inexpensive and plentiful, which lowers the setup cost and makes modifications possible. It is the only practical or economical way to produce very large castings. The lead time of sand casting is also short, making it a viable process for short production runs.
Sand casting’s versatility makes it a manufacturing option across a wide array of industries. It can produce medical equipment, automobile parts, electronic equipment, gas tanks, and engine blocks, and more.
Sand casting creates highly porous, textured metals. The shrinkage and rough surface finish also lower the dimensional accuracy of parts. This results in a low-strength final product that requires time-consuming post-processing to achieve a higher quality finish.
In order to choose the right industrial metal casting process, several factors must be considered. We’ve created this comparison table to help you compare die casting, investment casting, and sand casting in terms of types of metals, production volume, costs, production time, part complexity, and for which industries they are generally used.
Die Casting | Investment Casting | Sand Casting | |
---|---|---|---|
Compatible metals | Aluminum, copper, lead, magnesium, zinc | Most metals | Most metals |
Production volume | High volume | Low to high volume | One-off to medium volume |
Unit costs | Low | Moderate to high | Moderate |
Tooling costs | High | Moderate | Low |
Cycle time | Rapid | Long | Moderate |
Industries | Automotive, aerospace, consumer products, furniture, power tools | Automotive, aerospace, jewelry, medicine, dentistry, art | Automotive, aerospace, industrial equipment, electronics, consumer products |
3D printed jewelry ring pattern and cast metal part.
Engineers, designers, jewelers, and hobbyists can capitalize on the speed and flexibility of 3D printing by combining castings processes like indirect investment casting, direct investment casting, pewter casting, and sand casting with 3D printed patterns or casting metal into 3D printed molds. Cast metal parts using 3D printed rapid tooling can be produced in a fraction of the time invested in traditional casting and at a significantly lower cost than metal 3D printing.
Stereolithography (SLA) 3D printers offer high precision and a broad material library that is well-suited for casting workflows and can produce metal parts at a lower cost, with greater design freedom, and in less time than traditional methods.
Webinar
In this webinar, we will look at how desktop stereolithography (SLA) 3D printers are being used to directly print patterns, how to work with SLA patterns for investment casting, and how the benefits of generative design are increasing the demand for printed patterns.
Watch the Webinar Now
A 3D printed mold in Clear Resin for wax injection.
The process of making patterns from molds or tooling is referred to as indirect investment casting because it requires creating molds for producing the patterns in addition to final investment molds.
Rigid molds for wax (often referred to as tools) are commonly fabricated by machining aluminum or steel. Machined metal molds cost thousands of dollars to produce and take weeks of machining and polishing work before first shots can be run and pattern parts evaluated within a casting process.
With 3D printing, manufacturers can directly 3D print the mold for their pattern using materials like High Temp Resin or Rigid 10K Resin, resins with high-temperature resistance. For an optimal surface finish of molded parts, treat the interior surfaces of the mold by sanding and polishing for a smooth look, or bead blasting if a uniform matte look is desired. To ensure the final cast parts are dimensionally accurate, compensate for shrinkage by scaling up the printed mold. The exact shrinkage of the wax and the casting process can be obtained from supplier specifications.
3D printed molds for metal casting shorten the time between concept and first tests to a matter of days because manufacturers can directly print the tooling necessary for running and evaluating parts.
While molded pieces must follow design rules for moldability (e.g., no undercuts, draft is beneficial, etc.), you can achieve increased pattern complexity by using assembly jigs to combine multiple components into a single structure.
White Paper
Download our white paper to learn about six moldmaking processes that are possible with an in-house SLA 3D printer, including injection molding, vacuum forming, silicone molding, and more.
Download the White Paper
3D printed jewelry patterns and metal casted rings.
Direct investment casting is a version of investment casting where the process moves directly from pattern creation to surrounding the pattern with investment material. It is ideal for producing parts with geometries that are too complex to be molded or for parts with extensive undercuts and fine surface texture details, where molding is possible but carries high tooling costs.
Traditionally, patterns for direct investment casting are carved by hand or machined if the part is a one-off or expected to be only a handful of units. With 3D printing, however, manufacturers can directly print the patterns, removing the design and time constraints common in other processes.
With 3D printing, engineers, designers, and jewelers can direct 3D print patterns in order to achieve shorter lead times and geometric freedom that exceeds the design for manufacturability constraints of molding processes. Formlabs developed a range of castable materials suitable for direct investment casting, in particular for the jewelry industry.
White Paper
The way jewelers work is changing, and castable photopolymer resins are leading the way. In this guide, learn how to cast fine jewelry pieces 3D printed on Formlabs printers.
Download the White Paper
Sample part
See and feel Formlabs quality firsthand. We’ll ship a free sample part to your office.
Request a Free Sample Part
Grey Resin printed pattern and finished aluminum casting from an open-faced sand mold.
Similar to investment casting, 3D printing can be used to create patterns for sand casting.
In comparison to traditional materials like wood, 3D printing allows manufacturers to create complex shapes and go straight from digital design to casting.
Metal Miniatures made with pewter casting and 3D printing.
Pewter is a malleable metal alloy with a low melting point that can be used for making fully metal objects for decorative applications such as detailed metal miniatures, jewelry, scale models, and replicas of antiques.
With recent developments in temperature-resistant 3D printing materials, like High Temp Resin for Formlabs SLA 3D printers, it’s now possible to 3D print molds for direct pewter casting.
There are two options for mold designs: a sacrificial or pull-apart mold. In a sacrificial mold, there is a shell designed to be broken apart in the process. Pull-apart molds function with separate halves so the mold can be reused.
Compared to directly printing metal, the casting pewter in 3D printed molds offers significantly better detail and surface finish at a small fraction of the cost. Compared to wax casting, directly 3D printing a mold has fewer steps and requires less manual effort, while preserving the most possible detail.
Webinar
Watch our webinar to learn how moldmaking using 3D printed masters and reusable or sacrificial molds can allow you to produce parts in porcelain, precious and non-precious metals, silicone and biocompatible flexible materials, and more.
Watch the Webinar Now
Jewelry investment casting process with 3D printed patterns.
Businesses looking to boost design freedom or cut costs and lead times have a strong solution in metal casting with 3D printing.
Certain types of complex metal castings, such as large shapes with cross-sections and pieces with multiple cores, are difficult to create using traditional metal casting methods. 3D printing allows manufacturers to produce these complex designs. For example, jewelers can create intricate and custom designs that might be impossible without a 3D printed pattern.
3D printing also eliminates reliance on multiple machines or service providers to create parts. Instead, companies just need a digital file, a 3D printer, and printing material. This can cut costs and waste, as all the material used goes into the final product.
Finally, the combination of 3D printing with metal casting can cut costs and lead time. Rather than waiting weeks for expensive tooling before being able to cast a final product, a 3D printer can create a pattern or mold in hours.
Metal casting combined with 3D printing help companies quickly and efficiently create metal parts. With a Formlabs SLA 3D printer, you can expedite the metal casting process and cut costs along the way.
Learn more about the Form 3 desktop SLA 3D printer and request a free sample part to evaluate the quality firsthand.
See the Form 3Request a Free Sample Part
Producing low-cost cast metal parts using 3D printing
Learn how castable FDM prints can be used to produce low-cost metal parts via investment casting.
Using 3D printing to produce metal parts
DMLS
DMLS is a powder bed fusion technology that is used to produce metal parts to a high level of dimensional accuracy. The additive nature of 3D printing means that very complex designs are able to be created. The design freedom offered by DMLS has seen it adopted by many industries (automotive and aerospace) where weight optimisation and performance are critical (the cost of operating a commercial aircraft is roughly €1000/kg meaning any weight saving result in significant savings in operation costs). This has seen these industries willing to justify the high per part cost of DMLS based on the cost savings of producing complex lighter parts. It is always advisable to compare the cost of using a 3D printing service provider and buying your own metal 3D printer.
DMLS can produce parts from a large range of metals including aluminium and stainless steel as well as exotic biocompatible materials used in dentistry and medical industries like titanium. The main limitations of DMLS are the high cost, small build size and long lead times compared to other 3D printing technologies. Parts also require support material to limit the likelihood of distortion and warping occurring and this must also be removed after printing further increasing lead time and cost.
A large number of metal crown and bridge copings printed in a single print(image courtesy of Renishaw)
Investment casting
The investment casting process traditionally uses wax patterns to produce molds for casting, as it has a very clean burn-out with no residues. The image below presents the process.
For low-run investment casting, patterns are traditionally machined from a wax block via CNC. Alternatively, for larger series, a die is machined and the patterns are created by casting the wax using the die. Tooling is a very expensive investment with production of the dies often taking a very long time (2 - 6 weeks).
3D printing is now regularly used in conjunction with a range of investment casting applications to produce patterns from castable materials. Castable 3D prints are commonplace in the dental and jewelry industries and are generally produced via the SLA / DLP printing process. This is a vat-photopolymerization technology that is able to produce parts with a very smooth surface and extremely fine details. The main limitation around SLA is the printer build volume size, or the high cost involved for larger patterns. For larger metal parts, castable FDM offers a cost effective, rapid solution.
Small, intricates design are perfect for SLA investment casting.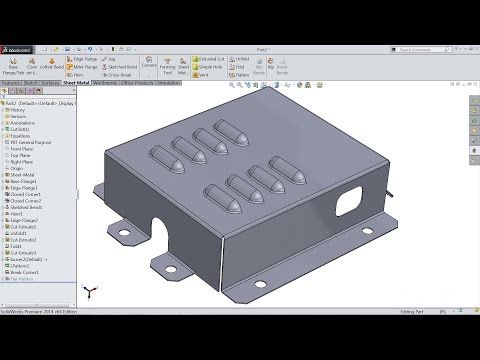
(image courtesy of Formlabs)
For larger metal parts, castable FDM offers a cost effective, rapid solution.
Ready to transform your CAD file into a custom part? Upload your designs for a free, instant quote.
Get an instant quoteCasting on models printed on a 3D printer / Sudo Null IT News
Jeshua Lacock writes:
This page describes my first and successful attempt to cast aluminum parts directly from 3D printed PLA models. The process is almost identical to wax casting, but instead of burning the wax, I burned the PLA plastic (bio-plastic)
I needed aluminum parts to mount the focus lens for my 150W CO2 cutting laser. These parts had to be adjustable and had to be mounted on a frame assembled from scrap aluminum found. nine0004
I get excited when I think about being able to do it all in one day: concept -> design -> 3D printing -> finished metal casting
Step one - detail design.
I used the amazingly powerful OpenSCAD to quickly design the parts I needed. OpenSCAD reads the script in its own language to draw the 3D model. In my case, I just drew a shape in Adobe Illustrator and converted it to OpenSCAD format using the Inkscape plugin. nine0004
Here is the design of the second part. I drew two shapes in Illustrator and gave them depth in OpenSCAD. Very fast and easy. Now I have 3D models, it's time to print them!
The process of printing the first part on my Ultimaker (fast shot).
The resulting part is in hand.
Printing the second part.
Second printed part in hand.
Checking how both parts fit together. nine0004
Sprues and air ducts are attached, everything is ready for pouring the molding mass.
Molding mass - 50% fine sand and 50% gypsum.
After adding water and mixing thoroughly, I used a home vacuum food sealer to get rid of air bubbles in the sand.
The second piece is ready to be molded!
The first detail is poured!
The part is completely immersed in the mixture - I hope everything works out. nine0004
Both molds are set to dry.
After a couple of hours, I placed the molds in the oven on medium heat.
My new little oven. Heats up quickly for 10 pounds (~4.5kg) or less of molten aluminium. 2 times more when it flares up.
Forms are good and well done. Once heated to about 1200F (650C), the plastic doesn't stand a chance. After removing, I blew out the molds with compressed air to blow out any remaining ash. nine0004
The still hot molds are placed in dry sand. It serves several purposes:
- additional support since the molds are quite fragile
- insulates molds and retains heat when cured
- will allow me to install sprue extensions (see below). The expander gives extra pressure to the casting and also acts as a riser that stays molten and provides extra metal while the casting solidifies and contracts
My sprues are just cut aluminum cans. Works great!
After melting aluminum with a small addition of copper in the crucible, removing the slag - it's time to pour!
So cool! It looks like the forms filled out perfectly. Now the hard part is to wait long enough for the metal to cool for me to know if the parts are good or not.
Excellent! The details are straight from the sand, only the sprues and air ducts are cut off. nine0004
I cut the center hole with a small 14mm tap. Everything seems to fit together nicely.
I like the details on the casting - you can see all the lines from the 3D printing, their width is only 0.2mm.
The moment of truth - do they fit the frame? YES! I made a plastic part to fit the frame perfectly, then printed it again at 102% size increase. When aluminum cools, it shrinks by exactly 2%.
The center of the black nipple is where the focused CO2 laser beam will come out. Tube - for compressed air to blow off smoke in the path of the laser (the so-called air-assist). nine0004
Looks like I can put this to good use!
The black hole in the center is a focusing lens.
I'm so happy that the parts fit the frame perfectly without any additional processing and even without a file!
This is the first test of my laser. Approximately 60% power. Burns wood instantly.
Translator:
Jeshua Lacock has made a wonderful, actually step-by-step guide on how to cast aluminum from 3D printed plastic models. For those interested in the practical aspect, there are many recipes on the site instructables.com on how to make an aluminum casting furnace. Even from a coffee can! nine0004
Create metal castings with 3D printed PLA molds
It was my first time experimenting with casting metal in PLA molds, for this project I used recycled aluminum scrap. In the past, I created two forms with 3D printer photogrammetry of two statues, since then I've been creating 3D printed statues of different sizes, but always wanted them to be made from a more durable material such as metal. Below is a brief description of the creation steps, but to see this method in more detail, watch the attached video showing the entire process from start to finish. When performing this project, please use all personal protective equipment presented in the video, remember safety above all else. nine0004
Note: This article is a translation.
Step 1: Full video on how to use PLA casting method
Step 2: Using the photogrammetry method to convert images into objects for 3D printing
I started by using the photogrammetry method, taking about 80 shots of each of the objects that I planned to cast in metal. The main software I worked with was Autodesk ReCap Photo. After completing the meshing and texturing phase, I did the final touches by removing additional backgrounds and cosmetic fixes on the surface of the object. The next step was to load the files into the Ultimaker Cura 3D printing software and test some real 3D prints. On the images, you can see the stages of preparing photogrammetry, both for the owl statue and for the weeping statue (aka the Legend of the Yogi Man - "Orang Malu"). nine0004
Step 3: 3D print two PLA statues for metal casting
I decided to experiment with 4 hollow 3D printed templates, 2 with 2mm shell thickness and 2 with 1mm shell thickness. I wanted to experiment to see if there would be a difference in the aluminum castings and my guess was correct: two 2mm aluminum castings didn't work, and the other two with 1mm wall thickness turned out much better, both not perfect but look great for the PLA test . I also learned that when casting in PLA molds it is important to expand the 3D print and add another 1cm of the base of the object as the PLA rises up after pouring molten aluminum and in my casting I lost 1cm of height compared to the original 3D print . nine0004
Step 4: PLA 3D prints for metal casting
I wasn't sure if a 1mm thick print would work without internal support, but to my surprise it worked out very well, I changed the settings in Cura for outer shell thickness and 0% infill for all four shapes.
Step 5: Use oil-bonded sand for Lost PLA sand casting
I used my very old but still usable Petrobond (oil soaked sand) and buried the four 3D shapes in the sand, then I used my mini soldering torch to make a hole in the base where the molten aluminum was to be poured , the idea was to try and remove some of the PLA in the hope that this would reduce some of the casting defects as the PLA floats when the molten aluminum is poured into the mold. nine0004
Step 6: Casting aluminum in a mold, successes and failures
I used my good old furnace and melted down some recycled aluminum scrap, after degassing and removing impurities, I poured molten aluminum into four sand mold cavities. After the aluminum had cooled down a bit, I removed the fresh sand castings and was surprised that my guess was correct, and the 1mm thick 3D molds were successful, while the 2mm thick ones were not.