3D printing guide for beginners
The Free Beginner's Guide - 3D Printing Industry
Welcome to 3DPI”s Beginner’s Guide to 3D Printing. Whether you are new to 3D printing technology or just looking to close a few knowledge gaps, we’re glad you stopped by. By now, most of us have heard, at some level, about the potential of 3D printing. But with this guide we are offering insights into the history and the reality of 3D printing — the processes, materials and applications — as well as measured thoughts on where it might be heading. We hope you’ll find this to be one of the most comprehensive 3D printing resources available, and that no matter what your skill level is, there will be plenty in here to meet your needs.
Are you ready? Let’s get started !
01 - Basics
3D Printing — also known as additive manufacturing — has been quoted in the Financial Times and by other sources as potentially being larger than the Internet. Some believe this is true. Many others urge that this is part of the extraordinary hype that exists around this very exciting technology area. So what really is 3D printing, who generally uses 3D printers and what for ?
Overview
The term 3D printing covers a host of processes and technologies that offer a full spectrum of capabilities for the production of parts and products in different materials. Essentially, what all of the processes and technologies have in common is the manner in which production is carried out layer by layer in an additive process which is in contrast to traditional methods of production involving subtractive methods or moulding/casting processes. Applications of 3D printing are emerging almost by the day, and, as this technology continues to penetrate more widely and deeply across industrial, maker and consumer sectors, this is only set to increase. Most reputable commentators on this technology sector agree that, as of today, we are only just beginning to see the true potential of 3D printing. 3DPI, a reliable media source for 3D printing, brings you all of the latest news, views, process developments and applications as they emerge in this exciting field. This overview article aims to provide the 3DPI audience with a reliable backgrounder on 3D printing in terms of what it is (technologies, processes and materials), its history, application areas and benefits
Introduction – What is 3D printing ?
3D Printing is a process for making a physical object from a three-dimensional digital model, typically by laying down many successive thin layers of a material. It brings a digital object (its CAD representation) into its physical form by adding layer by layer of materials.
There are several different techniques to 3D Print an object. We will go in further details later in the Guide. 3D Printing brings two fundamental innovations: the manipulation of objects in their digital format and the manufacturing of new shapes by addition of material.
Digital
+
Additive Manufacturing
Technology has affected recent human history probably more than any other field. Think of a light bulb, steam engine or, more latterly, cars and aeroplanes, not to mention the rise and rise of the world wide web. These technologies have made our lives better in many ways, opened up new avenues and possibilities, but usually it takes time, sometimes even decades, before the truly disruptive nature of the technology becomes apparent.
It is widely believed that 3D printing or additive manufacturing (AM) has the vast potential to become one of these technologies. 3D printing has now been covered across many television channels, in mainstream newspapers and across online resources. What really is this 3D printing that some have claimed will put an end to traditional manufacturing as we know it, revolutionize design and impose geopolitical, economic, social, demographic, environmental and security implications to our every day lives?
The most basic, differentiating principle behind 3D printing is that it is an additive manufacturing process. And this is indeed the key because 3D printing is a radically different manufacturing method based on advanced technology that builds up parts, additively, in layers at the sub mm scale. This is fundamentally different from any other existing traditional manufacturing techniques.
There are a number of limitations to traditional manufacturing, which has widely been based on human labour and made by hand ideology rooting back to the etymological origins of the French word for manufacturing itself. However, the world of manufacturing has changed, and automated processes such as machining, casting, forming and moulding are all (relatively) new, complex processes that require machines, computers and robot technology.
However, these technologies all demand subtracting material from a larger block whether to achieve the end product itself or to produce a tool for casting or moulding processes and this is a serious limitation within the overall manufacturing process.
For many applications traditional design and production processes impose a number of unacceptable constraints, including the expensive tooling as mentioned above, fixtures, and the need for assembly for complex parts. In addition, the subtractive manufacturing processes, such as machining, can result in up to 90% of the original block of material being wasted. In contrast, 3D printing is a process for creating objects directly, by adding material layer by layer in a variety of ways, depending on the technology used. Simplifying the ideology behind 3D printing, for anyone that is still trying to understand the concept (and there are many), it could be likened to the process of building something with Lego blocks automatically.
3D printing is an enabling technology that encourages and drives innovation with unprecedented design freedom while being a tool-less process that reduces prohibitive costs and lead times. Components can be designed specifically to avoid assembly requirements with intricate geometry and complex features created at no extra cost. 3D printing is also emerging as an energy-efficient technology that can provide environmental efficiencies in terms of both the manufacturing process itself, utilising up to 90% of standard materials, and throughout the products operating life, through lighter and stronger design.
In recent years, 3D printing has gone beyond being an industrial prototyping and manufacturing process as the technology has become more accessible to small companies and even individuals. Once the domain of huge, multi-national corporations due to the scale and economics of owning a 3D printer, smaller (less capable) 3D printers can now be acquired for under $1000.
This has opened up the technology to a much wider audience, and as the exponential adoption rate continues apace on all fronts, more and more systems, materials, applications, services and ancillaries are emerging.
02 - History
The earliest 3D printing technologies first became visible in the late 1980’s, at which time they were called Rapid Prototyping (RP) technologies. This is because the processes were originally conceived as a fast and more cost-effective method for creating prototypes for product development within industry. As an interesting aside, the very first patent application for RP technology was filed by a Dr Kodama, in Japan, in May 1980. Unfortunately for Dr Kodama, the full patent specification was subsequently not filed before the one year deadline after the application, which is particularly disastrous considering that he was a patent lawyer! In real terms, however, the origins of 3D printing can be traced back to 1986, when the first patent was issued for stereolithography apparatus (SLA). This patent belonged to one Charles (Chuck) Hull, who first invented his SLA machine in 1983. Hull went on to co-found 3D Systems Corporation — one of the largest and most prolific organizations operating in the 3D printing sector today.
3D Systems’ first commercial RP system, the SLA-1, was introduced in 1987 and following rigorous testing the first of these system was sold in 1988. As is fairly typical with new technology, while SLA can claim to be the first past the starting post, it was not the only RP technology in development at this time, for, in 1987, Carl Deckard, who was working at the University of Texas, filed a patent in the US for the Selective Laser Sintering (SLS) RP process. This patent was issued in 1989 and SLS was later licensed to DTM Inc, which was later acquired by 3D Systems. 1989 was also the year that Scott Crump, a co-founder of Stratasys Inc. filed a patent for Fused Deposition Modelling (FDM) — the proprietary technology that is still held by the company today, but is also the process used by many of the entry-level machines, based on the open source RepRap model, that are prolific today. The FDM patent was issued to Stratasys in 1992. In Europe, 1989 also saw the formation of EOS GmbH in Germany, founded by Hans Langer. After a dalliance with SL processes, EOS’ R&D focus was placed heavily on the laser sintering (LS) process, which has continued to go from strength to strength. Today, the EOS systems are recognized around the world for their quality output for industrial prototyping and production applications of 3D printing. EOS sold its first ‘Stereos’ system in 1990. The company’s direct metal laser sintering (DMLS) process resulted from an initial project with a division of Electrolux Finland, which was later acquired by EOS.
Other 3D printing technologies and processes were also emerging during these years, namely Ballistic Particle Manufacturing (BPM) originally patented by William Masters, Laminated Object Manufacturing (LOM) originally patented by Michael Feygin, Solid Ground Curing (SGC) originally patented by Itzchak Pomerantz et al and ‘three dimensional printing’ (3DP) originally patented by Emanuel Sachs et al. And so the early nineties witnessed a growing number of competing companies in the RP market but only three of the originals remain today — 3D Systems, EOS and Stratasys.
Throughout the 1990’s and early 2000’s a host of new technologies continued to be introduced, still focused wholly on industrial applications and while they were still largely processes for prototyping applications, R&D was also being conducted by the more advanced technology providers for specific tooling, casting and direct manufacturing applications. This saw the emergence of new terminology, namely Rapid Tooling (RT), Rapid Casting and Rapid Manufacturing (RM) respectively.
In terms of commercial operations, Sanders Prototype (later Solidscape) and ZCorporation were set up in 1996, Arcam was established in 1997, Objet Geometries launched in 1998, MCP Technologies (an established vacuum casting OEM) introduced the SLM technology in 2000, EnvisionTec was founded in 2002, ExOne was established in 2005 as a spin-off from the Extrude Hone Corporation and Sciaky Inc was pioneering its own additive process based on its proprietary electron beam welding technology. These companies all served to swell the ranks of Western companies operating across a global market. The terminology had also evolved with a proliferation of manufacturing applications and the accepted umbrella term for all of the processes was Additive Manufacturing (AM). Notably, there were many parallel developments taking place in the Eastern hemisphere. However, these technologies, while significant in themselves and enjoying some local success, did not really impact the global market at that time.
During the mid noughties, the sector started to show signs of distinct diversification with two specific areas of emphasis that are much more clearly defined today. First, there was the high end of 3D printing, still very expensive systems, which were geared towards part production for high value, highly engineered, complex parts. This is still ongoing — and growing — but the results are only now really starting to become visible in production applications across the aerospace, automotive, medical and fine jewellery sectors, as years of R&D and qualification are now paying off. A great deal still remains behind closed doors and/or under non-disclosure agreements (NDA). At the other end of the spectrum, some of the 3D printing system manufacturers were developing and advancing ‘concept modellers’, as they were called at the time. Specifically, these were 3D printers that kept the focus on improving concept development and functional prototyping, that were being developed specifically as office- and user-friendly, cost-effective systems. The prelude to today’s desktop machines. However, these systems were all still very much for industrial applications.
Looking back, this was really the calm before the storm.
At the lower end of the market — the 3D printers that today are seen as being in the mid range — a price war emerged together with incremental improvements in printing accuracy, speed and materials.
In 2007, the market saw the first system under $10,000 from 3D Systems, but this never quite hit the mark that it was supposed to. This was partly due to the system itself, but also other market influences. The holy grail at that time was to get a 3D printer under $5000 — this was seen by many industry insiders, users and commentators as the key to opening up 3D printing technology to a much wider audience. For much of that year, the arrival of the highly-anticipated Desktop Factory — which many predicted would be the fulfillment of that holy grail — was heralded as the one to watch. It came to nothing as the organization faltered in the run up to production. Desktop Factory and its leader, Cathy Lewis, were acquired, along with the IP, by 3D Systems in 2008 and all but vanished. As it turned out though, 2007 was actually the year that did mark the turning point for accessible 3D printing technology — even though few realized it at the time — as the RepRap phenomenon took root. Dr Bowyer conceived the RepRap concept of an open source, self-replicating 3D printer as early as 2004, and the seed was germinated in the following years with some heavy slog from his team at Bath, most notably Vik Oliver and Rhys Jones, who developed the concept through to working prototypes of a 3D printer using the deposition process. 2007 was the year the shoots started to show through and this embryonic, open source 3D printing movement started to gain visibility.
But it wasn’t until January 2009 that the first commercially available 3D printer – in kit form and based on the RepRap concept – was offered for sale. This was the BfB RapMan 3D printer. Closely followed by Makerbot Industries in April the same year, the founders of which were heavily involved in the development of RepRap until they departed from the Open Source philosophy following extensive investment. Since 2009, a host of similar deposition printers have emerged with marginal unique selling points (USPs) and they continue to do so. The interesting dichotomy here is that, while the RepRap phenomenon has given rise to a whole new sector of commercial, entry-level 3D printers, the ethos of the RepRap community is all about Open Source developments for 3D printing and keeping commercialization at bay.
2012 was the year that alternative 3D printing processes were introduced at the entry level of the market. The B9Creator (utilising DLP technology) came first in June, followed by the Form 1 (utilising stereolithography) in December. Both were launched via the funding site Kickstarter — and both enjoyed huge success.
As a result of the market divergence, significant advances at the industrial level with capabilities and applications, dramatic increase in awareness and uptake across a growing maker movement, 2012 was also the year that many different mainstream media channels picked up on the technology. 2013 was a year of significant growth and consolidation. One of the most notable moves was the acquisition of Makerbot by Stratasys.
Heralded as the 2nd, 3rd and, sometimes even, 4th Industrial Revolution by some, what cannot be denied is the impact that 3D printing is having on the industrial sector and the huge potential that 3D printing is demonstrating for the future of consumers. What shape that potential will take is still unfolding before us.
03 - Technology
The starting point for any 3D printing process is a 3D digital model, which can be created using a variety of 3D software programmes — in industry this is 3D CAD, for Makers and Consumers there are simpler, more accessible programmes available — or scanned with a 3D scanner. The model is then ‘sliced’ into layers, thereby converting the design into a file readable by the 3D printer. The material processed by the 3D printer is then layered according to the design and the process. As stated, there are a number of different types of 3D printing technologies, which process different materials in different ways to create the final object. Functional plastics, metals, ceramics and sand are, now, all routinely used for industrial prototyping and production applications. Research is also being conducted for 3D printing bio materials and different types of food. Generally speaking though, at the entry level of the market, materials are much more limited. Plastic is currently the only widely used material — usually ABS or PLA, but there are a growing number of alternatives, including Nylon. There is also a growing number of entry level machines that have been adapted for foodstuffs, such as sugar and chocolate.
How it Works
The different types of 3D printers each employ a different technology that processes different materials in different ways. It is important to understand that one of the most basic limitations of 3D printing — in terms of materials and applications — is that there is no ‘one solution fits all’. For example some 3D printers process powdered materials (nylon, plastic, ceramic, metal), which utilize a light/heat source to sinter/melt/fuse layers of the powder together in the defined shape. Others process polymer resin materials and again utilize a light/laser to solidify the resin in ultra thin layers. Jetting of fine droplets is another 3D printing process, reminiscent of 2D inkjet printing, but with superior materials to ink and a binder to fix the layers. Perhaps the most common and easily recognized process is deposition, and this is the process employed by the majority of entry-level 3D printers. This process extrudes plastics, commonly PLA or ABS, in filament form through a heated extruder to form layers and create the predetermined shape.
Because parts can be printed directly, it is possible to produce very detailed and intricate objects, often with functionality built in and negating the need for assembly.
However, another important point to stress is that none of the 3D printing processes come as plug and play options as of today. There are many steps prior to pressing print and more once the part comes off the printer — these are often overlooked. Apart from the realities of designing for 3D printing, which can be demanding, file preparation and conversion can also prove time-consuming and complicated, particularly for parts that demand intricate supports during the build process. However there are continual updates and upgrades of software for these functions and the situation is improving. Furthermore, once off the printer, many parts will need to undergo finishing operations. Support removal is an obvious one for processes that demand support, but others include sanding, lacquer, paint or other types of traditional finishing touches, which all typically need to be done by hand and require skill and/or time and patience.
04 - Processes
Stereolithography
Stereolithography (SL) is widely recognized as the first 3D printing process; it was certainly the first to be commercialised. SL is a laser-based process that works with photopolymer resins, that react with the laser and cure to form a solid in a very precise way to produce very accurate parts. It is a complex process, but simply put, the photopolymer resin is held in a vat with a movable platform inside. A laser beam is directed in the X-Y axes across the surface of the resin according to the 3D data supplied to the machine (the . stl file), whereby the resin hardens precisely where the laser hits the surface. Once the layer is completed, the platform within the vat drops down by a fraction (in the Z axis) and the subsequent layer is traced out by the laser. This continues until the entire object is completed and the platform can be raised out of the vat for removal.
Because of the nature of the SL process, it requires support structures for some parts, specifically those with overhangs or undercuts. These structures need to be manually removed.
In terms of other post processing steps, many objects 3D printed using SL need to be cleaned and cured. Curing involves subjecting the part to intense light in an oven-like machine to fully harden the resin.
Stereolithography is generally accepted as being one of the most accurate 3D printing processes with excellent surface finish. However limiting factors include the post-processing steps required and the stability of the materials over time, which can become more brittle.
DLP — or digital light processing — is a similar process to stereolithography in that it is a 3D printing process that works with photopolymers. The major difference is the light source. DLP uses a more conventional light source, such as an arc lamp, with a liquid crystal display panel or a deformable mirror device (DMD), which is applied to the entire surface of the vat of photopolymer resin in a single pass, generally making it faster than SL.
Also like SL, DLP produces highly accurate parts with excellent resolution, but its similarities also include the same requirements for support structures and post-curing. However, one advantage of DLP over SL is that only a shallow vat of resin is required to facilitate the process, which generally results in less waste and lower running costs.
Laser Sintering / Laser Melting
Laser sintering and laser melting are interchangeable terms that refer to a laser based 3D printing process that works with powdered materials. The laser is traced across a powder bed of tightly compacted powdered material, according to the 3D data fed to the machine, in the X-Y axes. As the laser interacts with the surface of the powdered material it sinters, or fuses, the particles to each other forming a solid. As each layer is completed the powder bed drops incrementally and a roller smoothes the powder over the surface of the bed prior to the next pass of the laser for the subsequent layer to be formed and fused with the previous layer.
The build chamber is completely sealed as it is necessary to maintain a precise temperature during the process specific to the melting point of the powdered material of choice. Once finished, the entire powder bed is removed from the machine and the excess powder can be removed to leave the ‘printed’ parts. One of the key advantages of this process is that the powder bed serves as an in-process support structure for overhangs and undercuts, and therefore complex shapes that could not be manufactured in any other way are possible with this process.
However, on the downside, because of the high temperatures required for laser sintering, cooling times can be considerable. Furthermore, porosity has been an historical issue with this process, and while there have been significant improvements towards fully dense parts, some applications still necessitate infiltration with another material to improve mechanical characteristics.
Laser sintering can process plastic and metal materials, although metal sintering does require a much higher powered laser and higher in-process temperatures. Parts produced with this process are much stronger than with SL or DLP, although generally the surface finish and accuracy is not as good.
Extrusion / FDM / FFF
3D printing utilizing the extrusion of thermoplastic material is easily the most common — and recognizable — 3DP process. The most popular name for the process is Fused Deposition Modelling (FDM), due to its longevity, however this is a trade name, registered by Stratasys, the company that originally developed it. Stratasys’ FDM technology has been around since the early 1990’s and today is an industrial grade 3D printing process. However, the proliferation of entry-level 3D printers that have emerged since 2009 largely utilize a similar process, generally referred to as Freeform Fabrication (FFF), but in a more basic form due to patents still held by Stratasys. The earliest RepRap machines and all subsequent evolutions — open source and commercial — employ extrusion methodology. However, following Stratasys’ patent infringement filing against Afiniathere is a question mark over how the entry-level end of the market will develop now, with all of the machines potentially in Stratasys’ firing line for patent infringements.
The process works by melting plastic filament that is deposited, via a heated extruder, a layer at a time, onto a build platform according to the 3D data supplied to the printer. Each layer hardens as it is deposited and bonds to the previous layer.
Stratasys has developed a range of proprietary industrial grade materials for its FDM process that are suitable for some production applications. At the entry-level end of the market, materials are more limited, but the range is growing. The most common materials for entry-level FFF 3D printers are ABS and PLA.
The FDM/FFF processes require support structures for any applications with overhanging geometries. For FDM, this entails a second, water-soluble material, which allows support structures to be relatively easily washed away, once the print is complete. Alternatively, breakaway support materials are also possible, which can be removed by manually snapping them off the part. Support structures, or lack thereof, have generally been a limitation of the entry level FFF 3D printers. However, as the systems have evolved and improved to incorporate dual extrusion heads, it has become less of an issue.
In terms of models produced, the FDM process from Stratasys is an accurate and reliable process that is relatively office/studio-friendly, although extensive post-processing can be required. At the entry-level, as would be expected, the FFF process produces much less accurate models, but things are constantly improving.
The process can be slow for some part geometries and layer-to-layer adhesion can be a problem, resulting in parts that are not watertight. Again, post-processing using Acetone can resolve these issues.
Inkjet
There are two 3D printing process that utilize a jetting technique.
Binder jetting: where the material being jetted is a binder, and is selectively sprayed into a powder bed of the part material to fuse it a layer at a time to create/print the required part. As is the case with other powder bed systems, once a layer is completed, the powder bed drops incrementally and a roller or blade smoothes the powder over the surface of the bed, prior to the next pass of the jet heads, with the binder for the subsequent layer to be formed and fused with the previous layer.
Advantages of this process, like with SLS, include the fact that the need for supports is negated because the powder bed itself provides this functionality. Furthermore, a range of different materials can be used, including ceramics and food. A further distinctive advantage of the process is the ability to easily add a full colour palette which can be added to the binder.
The parts resulting directly from the machine, however, are not as strong as with the sintering process and require post-processing to ensure durability.
Material jetting: a 3D printing process whereby the actual build materials (in liquid or molten state) are selectively jetted through multiple jet heads (with others simultaneously jetting support materials). However, the materials tend to be liquid photopolymers, which are cured with a pass of UV light as each layer is deposited.
The nature of this product allows for the simultaneous deposition of a range of materials, which means that a single part can be produced from multiple materials with different characteristics and properties. Material jetting is a very precise 3D printing method, producing accurate parts with a very smooth finish.
Selective Deposition Lamination (SDL)
SDL is a proprietary 3D printing process developed and manufactured by Mcor Technologies. There is a temptation to compare this process with the Laminated Object Manufacturing (LOM) process developed by Helisys in the 1990’s due to similarities in layering and shaping paper to form the final part. However, that is where any similarity ends.
The SDL 3D printing process builds parts layer by layer using standard copier paper. Each new layer is fixed to the previous layer using an adhesive, which is applied selectively according to the 3D data supplied to the machine. This means that a much higher density of adhesive is deposited in the area that will become the part, and a much lower density of adhesive is applied in the surrounding area that will serve as the support, ensuring relatively easy “weeding,” or support removal.
After a new sheet of paper is fed into the 3D printer from the paper feed mechanism and placed on top of the selectively applied adhesive on the previous layer, the build plate is moved up to a heat plate and pressure is applied. This pressure ensures a positive bond between the two sheets of paper. The build plate then returns to the build height where an adjustable Tungsten carbide blade cuts one sheet of paper at a time, tracing the object outline to create the edges of the part. When this cutting sequence is complete, the 3D printer deposits the next layer of adhesive and so on until the part is complete.
SDL is one of the very few 3D printing processes that can produce full colour 3D printed parts, using a CYMK colour palette. And because the parts are standard paper, which require no post-processing, they are wholly safe and eco-friendly. Where the process is not able to compete favourably with other 3D printing processes is in the production of complex geometries and the build size is limited to the size of the feedstock.
EBM
The Electron Beam Melting 3D printing technique is a proprietary process developed by Swedish company Arcam. This metal printing method is very similar to the Direct Metal Laser Sintering (DMLS) process in terms of the formation of parts from metal powder. The key difference is the heat source, which, as the name suggests is an electron beam, rather than a laser, which necessitates that the procedure is carried out under vacuum conditions.
EBM has the capability of creating fully-dense parts in a variety of metal alloys, even to medical grade, and as a result the technique has been particularly successful for a range of production applications in the medical industry, particularly for implants. However, other hi-tech sectors such as aerospace and automotive have also looked to EBM technology for manufacturing fulfillment.
05 - Materials
The materials available for 3D printing have come a long way since the early days of the technology. There is now a wide variety of different material types, that are supplied in different states (powder, filament, pellets, granules, resin etc).
Specific materials are now generally developed for specific platforms performing dedicated applications (an example would be the dental sector) with material properties that more precisely suit the application.
However, there are now way too many proprietary materials from the many different 3D printer vendors to cover them all here. Instead, this article will look at the most popular types of material in a more generic way. And also a couple of materials that stand out.
Plastics
Nylon, or Polyamide, is commonly used in powder form with the sintering process or in filament form with the FDM process. It is a strong, flexible and durable plastic material that has proved reliable for 3D printing. It is naturally white in colour but it can be coloured — pre- or post printing. This material can also be combined (in powder format) with powdered aluminium to produce another common 3D printing material for sintering — Alumide.
ABS is another common plastic used for 3D printing, and is widely used on the entry-level FDM 3D printers in filament form. It is a particularly strong plastic and comes in a wide range of colours. ABS can be bought in filament form from a number of non-propreitary sources, which is another reason why it is so popular.
PLA is a bio-degradable plastic material that has gained traction with 3D printing for this very reason. It can be utilized in resin format for DLP/SL processes as well as in filament form for the FDM process. It is offered in a variety of colours, including transparent, which has proven to be a useful option for some applications of 3D printing. However it is not as durable or as flexible as ABS.
LayWood is a specially developed 3D printing material for entry-level extrusion 3D printers. It comes in filament form and is a wood/polymer composite (also referred to as WPC).
Metals
A growing number of metals and metal composites are used for industrial grade 3D printing. Two of the most common are aluminium and cobalt derivatives.
One of the strongest and therefore most commonly used metals for 3D printing is Stainless Steel in powder form for the sintering/melting/EBM processes. It is naturally silver, but can be plated with other materials to give a gold or bronze effect.
In the last couple of years Gold and Silver have been added to the range of metal materials that can be 3D printed directly, with obvious applications across the jewellery sector. These are both very strong materials and are processed in powder form.
Titanium is one of the strongest possible metal materials and has been used for 3D printing industrial applications for some time. Supplied in powder form, it can be used for the sintering/melting/EBM processes.
Ceramics
Ceramics are a relatively new group of materials that can be used for 3D printing with various levels of success. The particular thing to note with these materials is that, post printing, the ceramic parts need to undergo the same processes as any ceramic part made using traditional methods of production — namely firing and glazing.
Paper
Standard A4 copier paper is a 3D printing material employed by the proprietary SDL process supplied by Mcor Technologies. The company operates a notably different business model to other 3D printing vendors, whereby the capital outlay for the machine is in the mid-range, but the emphasis is very much on an easily obtainable, cost-effective material supply, that can be bought locally. 3D printed models made with paper are safe, environmentally friendly, easily recyclable and require no post-processing.
Bio Materials
There is a huge amount of research being conducted into the potential of 3D printing bio materials for a host of medical (and other) applications. Living tissue is being investigated at a number of leading institutions with a view to developing applications that include printing human organs for transplant, as well as external tissues for replacement body parts. Other research in this area is focused on developing food stuffs — meat being the prime example.
Food
Experiments with extruders for 3D printing food substances has increased dramatically over the last couple of years. Chocolate is the most common (and desirable). There are also printers that work with sugar and some experiments with pasta and meat. Looking to the future, research is being undertaken, to utilize 3D printing technology to produce finely balanced whole meals.
And finally, one company that does have a unique (proprietary) material offering is Stratasys, with its digital materials for the Objet Connex 3D printing platform. This offering means that standard Objet 3D printing materials can be combined during the printing process — in various and specified concentrations — to form new materials with the required properties. Up to 140 different Digital Materials can be realized from combining the existing primary materials in different ways.
06 - Global Effects
Global Effects on Manufacturing
3D printing is already having an effect on the way that products are manufactured – the nature of the technology permits new ways of thinking in terms of the social, economic,environmental and security implications of the manufacturing process with universally favourable results.
One of the key factors behind this statement is that 3D printing has the potential to bring production closer to the end user and/or the consumer, thereby reducing the current supply chain restrictions. The customisation value of 3D printing and the ability to produce small production batches on demand is a sure way to engage consumers AND reduce or negate inventories and stock piling — something similar to how Amazon operates its business.
Shipping spare parts and products from one part of the world to the other could potentially become obsolete, as the spare parts might possibly be 3D printed on site. This could have a major impact on how businesses large and small, the military and consumers operate and interact on a global scale in the future. The ultimate aim for many is for consumers to operate their own 3D printer at home, or within their community, whereby digital designs of any (customizable) product are available for download via the internet, and can be sent to the printer, which is loaded with the correct material(s). Currently, there is some debate about whether this will ever come to pass, and even more rigorous debate about the time frame in which it may occur.
The wider adoption of 3D printing would likely cause re-invention of a number of already invented products, and, of course, an even bigger number of completely new products. Today previously impossible shapes and geometries can be created with a 3D printer, but the journey has really only just begun. 3D printing is believed by many to have very great potential to inject growth into innovation and bring back local manufacturing.
Potential Effects to the Global Economy
The use of 3D printing technology has potential effects on the global economy, if adopted world wide. The shift of production and distribution from the current model to a localized production based on-demand, on site, customized production model could potentially reduce the imbalance between export and import countries.
3D printing would have the potential to create new industries and completely new professions, such as those related to the production of 3D printers. There is an opportunity for professional services around 3D printing, ranging from new forms of product designers, printer operators, material suppliers all the way to intellectual property legal disputes and settlements. Piracy is a current concern related to 3D printing for many IP holders.
The effect of 3D printing on the developing world is a double-edged sword. One example of the positive effect is lowered manufacturing cost through recycled and other local materials, but the loss of manufacturing jobs could hit many developing countries severely, which would take time to overcome.
The developed world, would benefit perhaps the most from 3D printing, where the growing aged society and shift of age demographics has been a concern related to production and work force. Also the health benefits of the medical use of 3D printing would cater well for an aging western society.
07 - Benefits & Value
3D printing, whether at an industrial, local or personal level, brings a host of benefits that traditional methods of manufacture (or prototyping) simply cannot.
Customisation
3D printing processes allow for mass customisation — the ability to personalize products according to individual needs and requirements. Even within the same build chamber, the nature of 3D printing means that numerous products can be manufactured at the same time according to the end-users requirements at no additional process cost.
Complexity
The advent of 3D printing has seen a proliferation of products (designed in digital environments), which involve levels of complexity that simply could not be produced physically in any other way. While this advantage has been taken up by designers and artists to impressive visual effect, it has also made a significant impact on industrial applications, whereby applications are being developed to materialize complex components that are proving to be both lighter and stronger than their predecessors. Notable uses are emerging in the aerospace sector where these issues are of primary importance.
Tool-less
For industrial manufacturing, one of the most cost-, time- and labour-intensive stages of the product development process is the production of the tools. For low to medium volume applications, industrial 3D printing — or additive manufacturing — can eliminate the need for tool production and, therefore, the costs, lead times and labour associated with it. This is an extremely attractive proposition, that an increasing number or manufacturers are taking advantage of. Furthermore, because of the complexity advantages stated above, products and components can be designed specifically to avoid assembly requirements with intricate geometry and complex features further eliminating the labour and costs associated with assembly processes.
Sustainable / Environmentally Friendly
3D printing is also emerging as an energy-efficient technology that can provide environmental efficiencies in terms of both the manufacturing process itself, utilising up to 90% of standard materials, and, therefore, creating less waste, but also throughout an additively manufactured product’s operating life, by way of lighter and stronger design that imposes a reduced carbon footprint compared with traditionally manufactured products.
Furthermore, 3D printing is showing great promise in terms of fulfilling a local manufacturing model, whereby products are produced on demand in the place where they are needed — eliminating huge inventories and unsustainable logistics for shipping high volumes of products around the world.
08 - Applications
The origins of 3D printing in ‘Rapid Prototyping’ were founded on the principles of industrial prototyping as a means of speeding up the earliest stages of product development with a quick and straightforward way of producing prototypes that allows for multiple iterations of a product to arrive more quickly and efficiently at an optimum solution. This saves time and money at the outset of the entire product development process and ensures confidence ahead of production tooling.
Prototyping is still probably the largest, even though sometimes overlooked, application of 3D printing today.
The developments and improvements of the process and the materials, since the emergence of 3D printing for prototyping, saw the processes being taken up for applications further down the product development process chain. Tooling and casting applications were developed utilizing the advantages of the different processes. Again, these applications are increasingly being used and adopted across industrial sectors.
Similarly for final manufacturing operations, the improvements are continuing to facilitate uptake.
In terms of the industrial vertical markets that are benefitting greatly from industrial 3D printing across all of these broad spectrum applications, the following is a basic breakdown:
Medical and Dental
The medical sector is viewed as being one that was an early adopter of 3D printing, but also a sector with huge potential for growth, due to the customization and personalization capabilities of the technologies and the ability to improve people’s lives as the processes improve and materials are developed that meet medical grade standards.
3D printing technologies are being used for a host of different applications. In addition to making prototypes to support new product development for the medical and dental industries, the technologies are also utilized to make patterns for the downstream metal casting of dental crowns and in the manufacture of tools over which plastic is being vacuum formed to make dental aligners. The technology is also taken advantage of directly to manufacture both stock items, such as hip and knee implants, and bespoke patient-specific products, such as hearing aids, orthotic insoles for shoes, personalised prosthetics and one-off implants for patients suffering from diseases such as osteoarthritis, osteoporosis and cancer, along with accident and trauma victims. 3D printed surgical guides for specific operations are also an emerging application that is aiding surgeons in their work and patients in their recovery. Technology is also being developed for the 3D printing of skin, bone, tissue, pharmaceuticals and even human organs. However, these technologies remain largely decades away from commercialisation.
Aerospace
Like the medical sector, the aerospace sector was an early adopter of 3D printing technologies in their earliest forms for product development and prototyping. These companies, typically working in partnership with academic and research institutes, have been at the sharp end in terms or pushing the boundaries of the technologies for manufacturing applications.
Because of the critical nature of aircraft development, the R&D is demanding and strenuous, standards are critical and industrial grade 3D printing systems are put through their paces. Process and materials development have seen a number of key applications developed for the aerospace sector — and some non-critical parts are all-ready flying on aircraft.
High profile users include GE / Morris Technologies, Airbus / EADS, Rolls-Royce, BAE Systems and Boeing. While most of these companies do take a realistic approach in terms of what they are doing now with the technologies, and most of it is R&D, some do get quite bullish about the future.
Automotive
Another general early adopter of Rapid Prototying technologies — the earliest incarnation of 3D printing — was the automotive sector. Many automotive companies — particularly at the cutting edge of motor sport and F1 — have followed a similar trajectory to the aerospace companies. First (and still) using the technologies for prototyping applications, but developing and adapting their manufacturing processes to incorporate the benefits of improved materials and end results for automotive parts.
Many automotive companies are now also looking at the potential of 3D printing to fulfill after sales functions in terms of production of spare/replacement parts, on demand, rather than holding huge inventories.
Jewellery
Traditionally, the design and manufacturing process for jewellery has always required high levels of expertise and knowledge involving specific disciplines that include fabrication, mould-making, casting, electroplating, forging, silver/gold smithing, stone-cutting, engraving and polishing. Each of these disciplines has evolved over many years and each requires technical knowledge when applied to jewellery manufacture. Just one example is investment casting — the origins of which can be traced back more than 4000 years.
For the jewellery sector, 3D printing has proved to be particularly disruptive. There is a great deal of interest — and uptake — based on how 3D printing can, and will, contribute to the further development of this industry. From new design freedoms enabled by 3D CAD and 3D printing, through improving traditional processes for jewellery production all the way to direct 3D printed production eliminating many of the traditional steps, 3D printing has had — and continues to have — a tremendous impact in this sector.
Art / Design / Sculpture
Artists and Sculptors are engaging with 3D printing in myriad of different ways to explore form and function in ways previously impossible. Whether purely to find new original expression or to learn from old masters this is a highly charged sector that is increasingly finding new ways of working with 3D printing and introducing the results to the world. There are numerous artists that have now made a name for themselves by working specifically with 3D modelling, 3D scanning and 3D printing technologies.
- Joshua Harker
- Dizingof
- Jessica Rosenkrantz at Nervous System
- Pia Hinze
- Nick Ervinck
- Lionel Dean
- And many others.
The discipline of 3D scanning in conjunction with 3D printing also brings a new dimension to the art world, however, in that artists and students now have a proven methodology of reproducing the work of past masters and creating exact replicas of ancient (and more recent) sculptures for close study – works of art that they would otherwise never have been able to interact with in person. The work of Cosmo Wenman is particularly enlightening in this field.
Architecture
Architectural models have long been a staple application of 3D printing processes, for producing accurate demonstration models of an architect’s vision. 3D printing offers a relatively fast, easy and economically viable method of producing detailed models directly from 3D CAD, BIM or other digital data that architects use. Many successful architectural firms, now commonly use 3D printing (in house or as a service) as a critical part of their workflow for increased innovation and improved communication.
More recently some visionary architects are looking to 3D printing as a direct construction method. Research is being conducted at a number of organizations on this front, most notably Loughborough University, Contour Crafting and Universe Architecture.
Fashion
As 3D printing processes have improved in terms of resolution and more flexible materials, one industry, renowned for experimentation and outrageous statements, has come to the fore. We are of course talking about fashion!
3D printed accessories including shoes, head-pieces, hats and bags have all made their way on to global catwalks. And some even more visionary fashion designers have demonstrated the capabilities of the tech for haute couture — dresses, capes, full-length gowns and even some under wear have debuted at different fashion venues around the world.
Iris van Herpen should get a special mention as the leading pioneer in this vein. She has produced a number of collections — modelled on the catwalks of Paris and Milan — that incorporate 3D printing to blow up the ‘normal rules’ that no longer apply to fashion design. Many have followed, and continue to follow, in her footsteps, often with wholly original results.
Although a late-comer to the 3D printing party, food is one emerging application (and/or 3D printing material) that is getting people very excited and has the potential to truly take the technology into the mainstream. After all, we will all, always, need to eat! 3D printing is emerging as a new way of preparing and presenting food.
Initial forays into 3D printing food were with chocolate and sugar, and these developments have continued apace with specific 3D printers hitting the market. Some other early experiments with food including the 3D printing of “meat” at the cellular protein level. More recently pasta is another food group that is being researched for 3D printing food.
Looking to the future 3D printing is also being considered as a complete food preparation method and a way of balancing nutrients in a comprehensive and healthy way.
Consumers
The holy grail for 3D printing vendors is consumer 3D printing. There is a widespread debate as to whether this is a feasible future. Currently, consumer uptake is low due to the accessibility issues that exist with entry level (consumer machines). There is headway being made in this direction by the larger 3D printing companies such as 3D Systems and Makerbot, as a subsidiary of Stratasys as they try to make the 3D printing process and the ancillary components (software, digital content etc) more accessible and user-friendly. There are currently three main ways that the person on the street can interact with 3D printing tech for consumer products:
- design + print
- choose + print
- choose + 3D printing service fulfillment
09 - Glossary
3DP 3D Printing
ABS Acrylonitrile Butadiene Styrene
AM Additive Manufacturing
CAD / CAM Computer-aided design / Computer-aided manufacturing
CAE Computer-aided engineering
DLP Digital Light Processing
DMD Direct Metal Deposition
DMLS Direct Metal Laser Sintering
EBM Electron Beam Melting
EVA Ethylene Vinyl Acetate
FDM Fused Deposition Modelling (Trademark of Stratasys)
FFF Freeform Fabrication
LENS Laser Engineering Net-Shaping (Trademark of SNL, licensed to Optomec)
LS Laser Sintering
PLA Polylactic Acid
RE Reverse Engineering
RM Rapid Manufacturing
RP Rapid Prototyping
RT Rapid Tooling
SL Stereolithography
SLA Stereolithography Apparatus (Registered Trademark of 3D Systems)
SLM Selective Laser Melting
SLS Selective Laser Sintering (Registered Trademark of 3D Systems)
STL / . stl Stereo Lithograpic
A Beginner's Guide to 3D Printing
3D printing is an additive manufacturing process that uses thin layers of filament (in most cases, plastic) to create a physical object from a three-dimensional model. A digital file creates the model which eventually transfers to the printer. The 3D printer creates thin layers, one on top of another, until a 3D printed object is formed. 3D printing also allows the production of models of more complex shapes with less material than traditional manufacturing techniques.
Research shows that 3D printing was first introduced in the ’70s. It was not until 1980 that early additive manufacturing equipment and materials were developed. Hideo Kodama initiated a patent for this technology but, unfortunately, never commercialized it. In the ’90s 3D printing began to attract attention from technologies around the world. These years also saw the invention of fully functional human organs for transplants in young patients using 3D printed methods covered with particles and cells from their very own body. It was a major success for the medical industry.
Despite these advancements, 3D printing had limited functional productions until the 2000s, when additive manufacturing gained popularity. Additive Manufacturing is the process of adding materials together to produce an item. The procedure of additive manufacturing is in stark contrast to the concept of subtractive manufacturing. Subtractive manufacturing is the process of removing material by carving out a surface to create an object. This process also produces a great deal of material waste. In this regard, the term 3D printing still refers more to technologies that use polymer materials and, additive manufacturing refers more to metalworking. But by the early 2010s, the terms of these two processes were used in popular language across the market, media, companies, and manufacturers.
Around 2008 the first self-replicating 3d printer model was created. That means a 3D printer was able to recreate itself by printing its parts and components. This enabled users to produce more printers for others. Studies show that later the same year, a person successfully walked with a 3D printed prosthetic leg fully printed in one piece. Then in the 2010’s the additive processes matured, and 3D printing work began to create objects layer by layer. In 2012, with the addition of plastic and other various materials for 3D printing, several authors began to think that 3D printing could be important for a developing world.
During the following years, more applications for 3D printing have emerged, including the world’s first aircraft. Makers using 3D printers agree that this method is faster and cheaper compared to traditional methods and are ideal for those who need rapid prototyping (RP). Terms such as desktop manufacturing, rapid manufacturing, and rapid prototyping have since become synonymous with 3D printing.
The market offers a wide variety of 3D printers. Sophisticated machines are expensive, but there are also more affordable models available with high-quality printing and features. 3D printing also offers easy-to-use desktop printers, which are increasingly popular among schools and engineers.
In a shell, 3D printing works by blending layers of material to build an object. In this process, the 3D printer works with the direction of a computer 3D modeling software that regulates the process with high precision and exactness.
The 3D printing manufacturing includes several types of manufacturing technologies, all these work in the same way by creating models’ layer by layer essentially. Each one of these types of 3D printing manufacturing processes may utilize a different type of material, finish, and cost.
Some of the most common and utilized types of technologies are FDM, SLS, SLM, SLA, and DLP. Below read on a summary of each one of these technologies.
Let us start with the most common of these, the FDM or Fused Deposition Modelling, this is a trading name given by Stratasys. Even though this concept has been around since the ’90s, a lot of 3D printers since 2009 starting to utilize this process. This technology is also known as FFF (Fuse Filament Fabrication). In this type of process, several layers are aligned together until a shape is formed, by melting plastic that is deposited via a heated extruder. The most common materials used or filaments of this type of process are ABS and PLA.
Another SLS or Selective Laser Sintering uses a laser to sinter powdered plastic material and turn this into a solid model. Normally, this type of technology is a popular choice due to the rapid ability to create prototypes and small-batch manufacturing.
SLM or Selective Laser Melting uses a high-power density laser to melt and fuse metallic types of powder. With his type of technology, the metal material can be fully melted into a solid 3D model. This process also allows for the shape to be created layer by layer and create parts that cannot be easily cast with other conventional methods. The file is sliced into layers on a CAD computer software, normally and .STL file, and then it is loaded onto a file preparation software, then the material is melted using a high- power laser beam until a part is complete.
Continuing SLA or Stereolithography creates parts with high levels of detail, smooth surfaces, flawless finishes, and quality. This type of technology is widely used for applications on the mechanical industry and models.
Finally, DLP or Digital Light Processing is a technique similar to SLA that cures the resin materials by using light through a light projector screen. Because of the light usage, an entire layer can be built at once making this process relatively faster but recommendable for low-volume production runs of mostly plastic parts.
How 3D Printing Works 1. Create a CAD (Computer-Aided Design) fileThe first step to creating a 3D printed object is creating a virtual design with computer software or a 3D scanner. On this, the exact dimensions of the object to build are simulated to see how this will look like when finishing the 3D printing. When designing a 3D object utilizing CAD, fewer errors may result while printing, and fortunately, these can be corrected before the process. There is also another way of creating an object manually, like sculpting where a 3D scanner is needed to collect the data, shape, and appearance of the desired object.
Once the design is being created, the next thing is to convert the file into a format that can be read by the 3D printer. One of the most common files used is STL (standard tessellation language). STL files may sometimes create a larger file due to the number of surfaces. There is also another option of a file format used named AMF, Additive Manufacturing File format that stores information more conveniently.
3. Manipulate the STL fileOnce the STL file is created, and ready to be sent to the 3D printer, the orientation and size for the object to be printed must be set. STL files also allow us to repair any inconsistencies in the original.
Once the digital file is ready to be printed, all materials need to be ready as well to start the printing process. Once the STL file is ready, then it must be processed by a slicing software that aids in the 3D printing process by converting the object into layers and provides the instructions that later will be received by the 3D printer.
5. Build the objectOnce all the mentioned parameters are ready, the printing process can begin. Some printers may take some time to create the final product as this depends on how complex is the object to print. Many printers have high-end capabilities and print faster. When the process begins, the layers start to build the object with an incomparable resolution using a special measure of micrometers. For instance, the thickness of a typical layer is about 100 micrometers.
6.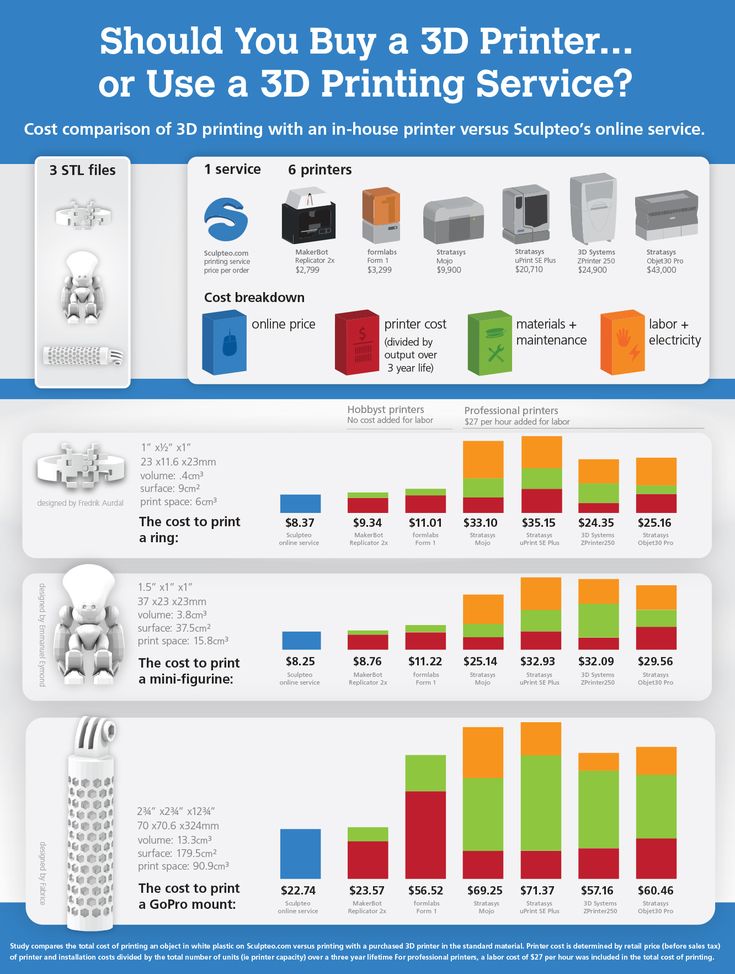
Once the object is ready, this must be handled very cautiously. For instance, putting gloves to handle the printed item is recommendable, finally, brush off any residual powder to clean up the piece. One of the advantages of 3D printing is that a piece can be made within hours, compared to traditional processes of manufacturing, this is very convenient and shows faster results.
How does an FFF 3D Printer Work?Fused Filament Fabrication (FFF), is also known under the trademarked term Fused Deposition Modeling (FDM). This technology was invented after SLA (Stereolithography) and SLS (Selective Laser Sintering) techniques were present. The term FFF was initially used as an unconstrained alternative given the fact that FDM is a trademarked term.
To begin with, an extrusion heated nozzle moves over a built platform, at the same time releases molten plastic, then this begins to deposit the thermoplastic material in thin layers, one on top of another onto a print bed, which is where eventually the 3D printed object is formed. The nozzle and the printed bed move while at the same time the plastic is being extruded. In this process, the slicing software is crucial due to this being the one that separates the design into different layers for 3D printing optimization.
3D printing uses a wide range of different variations of materials such as pastes, raw materials, and thermoplastics or filaments, being these the most used and come in different colors, thickness, and sizes to fit the purpose of the 3D printing model. Filament materials used for extrusion include thermoplastics, ABS, PLA, HIPS, TPU, ASA, PETG, PLA, etc.
What can be 3D Printed?3D printing has revolutionized the way models and prototypes are being created for the industry. The idea of rapid prototyping (RP) allows the creation of products usually within hours of days rather than weeks when traditional methods are used. With 3D printing, almost every object you can think of can be printed.
According to Statista, the worldwide market for 3D printing products and services is anticipated to exceed 40 billion U. S. dollars by 2024. This source states that this industry is expected to expand to an annual growth rate of 26.4 percent between 2020 and 2024.
3D printing can create a wide range of applications. Every day, new materials and applications are being discovered and therefore, more companies are relying on this method for quicker prototyping and production of items, including the fact that they already have their printers.
3D printing is actively involved across many important industrial organizations with a significant impact on product development, research, education, and more, and is promising to transform almost every industry as we currently know it.
3D Printing in the Consumer Goods IndustryMany companies and retailers are recurring to the usage of 3D printing due to its significant value on the commercial chain. They can customize and design their products in a quicker manner and keep up with the ever-changing consumer market. By producing pieces faster, they are also able to put their products rapidly in the market.
Some companies have used 3D printing to produce eyewear, footwear, lighting design, furniture, and more. Among the brands that have already produced athletic shoes are Nike and Adidas. In an article published by Nike at news.nike.com, they mention how Nike Flyprint is the first 3D printed textile upper-performance footwear. Nike Flyprint uppers are produced through SDM (solid deposit modeling).
Another application is 3D printing in jewelry. According to SmarTech the industry value of precious metals for additive manufacturing is expected to reach $1.8 billion worldwide by 2028. A famous Australian company Boltenstern, has launched a 3D printed jewelry line recently.
3D Printing in the Medical IndustryIn the medical field, 3D printing has a lot to contribute. While donors are difficult to find, in this video published by Marketwatch, the Rochester Institute of Technology’s engineering department is researching new 3D printing techniques for health-care applications such as the capability to generate organs that can be acceptable to the recipient. Allied Market Research shows that the 3D printing market for healthcare is expected to grow at $2.3 billion by 2020.
With the rapid advancement of flexible manufacturing and innovations, 3D printing is now widely implemented for medical purposes, such as implant designs, surgical planning and training, and prosthetics. See here some articles of 3D printing for medical applications, including a most recent case study of how people are using 3D printing to produce masks to fight COVID-19.
With the rapid advancement of flexible manufacturing and innovations in Biomedical fields, 3D printing is now widely implemented for medical purposes, such as implant designs, surgical planning and training, and prosthetics. You can 3D print with thermoplastics like Polycarbonate, semi-flexible plastics, ABS, which is strong and weather resistant or PLA (Polylactic Acid), which will biodegrade over time, even inside a human body. In this case, 3D printing is used in the field of radiotherapy is used to create custom devices for beam range modulation, 3D Conformal Radiation Therapy (3D CRT), or Brachytherapy application.
In this case, spinal surgeries see Increased success rate with 3D printed guides. The Bengbu Hospital is the top-grade hospital in the Anhui Province. Since the end of 2013, Director Niu launched 3D printing application research for vertebrae in the clinical field.
Here is another case, where 3D printing has reduced costs and help the creation of prosthetic hands. Founder Mike Li worked in the IT industry up until 3 years ago when he was inspired by a video that highlighted a unique use of 3D printing for children’s prosthetics. Motivated to apply medical 3D printing for prosthetics to help others, he and other local makers volunteered their time to create and customize prosthetics for patients.
3D Printing in the Automotive Industry3D printing is also transforming the automotive industry, evolving from printing relatively simple prototypes of low production parts to 3D printing entire cars. In-car auto designs, auto parts can also be created using 3D printing. Sometimes, a scale small model is printed to gauge scale before the assembly process. This technique also helps the industry by producing rapid prototypes and reducing money and time for production. Some other automotive companies are dedicated to creating customized auto parts for special model cars. Read here more case studies about 3D printing in the automotive industry.
In the aerospace industry, 3D printing has remarkable uses as well. To name a few, Airbus is utilizing 3D printing technology to create plastic parts on commercial A310 and A350 XWB test aircraft. In this video, metal parts for wing slats, a section of the tail wing and door hinges are claimed to be produced by this company. The development and manufacturing of potential parts using 3D printing can be conceived as lighter, stronger, and with 70% less time to make it and 80% less expensive compared to others. Aside from this, Airbus also mentions how 3D printing contributes to the environment as it has reduced up to 95% of its metal waste.
Research shows that the market for 3D printing dental applications is expected to grow significantly. Dental 3D printing applications include the creation of crowns, aligners, bridge models, retainers, and even orthodontic models. Read here about Dental 3D printing in Orthodontic models.
3D Printing for ProstheticsThe impact of 3D printing on the medical field has made positive advancements such as fast processing times, low costs, and the ability to create efficient prototypes and parts that require customization, such as 3D printed implants and prosthetics. 3D printing is producing hands, feet, legs, and more.
Albert Fung, a talented biology illustrator from Canada, first designed a CAD template for the initial prosthetic. Using this as a base, he and his team were able to optimize the model for each patient’s situation.
An organization named e-NABLE is currently doing work in this area. Albert Fung and Dr. Choi created five versions of the initial prosthetic design and optimized the design to accommodate individuals in Sierra Leone within one year. Read here a 3D Printing prosthetics case study.
In this field, 3D printing allows us to quickly create an architectural model, and this is ideal because a physical model is much favored than a computer presented a model on the screen. Any architectural application can rapidly create scale models in a faster and cheaper way now. There are also other astonishing applications of 3D printing in the architectural industry, to name a few it is possible to create entire buildings and urban structures. In Madrid, Spain the first 3D pedestrian bridge was printed. This structure crosses a stream in Castilla-La Mancha Park in Alcobendas, Madrid. The structure is printed using micro-reinforced concrete and measures 12 meters in length and 1.75 wide.
3D Printing in Archeology3D printing for museums and archeology is helping with the reproduction of exact copies of artifacts that can travel the world to help researches in their developments. Archeological pieces can also be scanned and created for students to do research. This technology is widely used by museums because ancient pieces are at a high risk of being broken or damaged when transported and by the usage of scanning and 3D printing, restoration is possible. This including fossil reconstruction.
Although restoration is a field dedicated to preserving the past, some sculptors are turning to 3D printing to help facilitate their restoration work. A great example of this is the Scuola di Alta Formazione (SAF) of the Instituto Superiore per la Conservazione ed il Restauro (ISCR). This institute is the leader of the restoration of masterpieces of the Italian heritage. Teachers at the institute decided to use 3D scanning and 3D printing with excellent results for their restoration projects. Read here 3D printing in the restoration of Italian classical art.
Another example of this great use is a project called “Elastic Minds” by the MOMA, the Museum of Modern Art in New York, the artists were using 3D printing in a project to create art and furniture such as chairs at a complete scale. In this video about the exhibition, pieces of furniture were created by sketches in the air with laser technology and then a camera scans this to capture the movement and captures this as a drawing that then is sent into a 3D printer machine.
In forensics, the usage of 3D printing is creating a breakthrough in solving cold case files, by printing skulls, shoe prints instantly, and more. Daryl Ricketts is a forensic anthropologist and a professor of anthropology at Indiana University that uses 3D printing for education and research purposes. He uses the resources of 3D printing to create forensic pieces for his students. By using CT scans, fetal specimens, fetal skeletons to do virtual autopsies. He also uses facial 3D printing for facial reconstruction from different hominids.
Furthermore, at the University of South Florida, forensic artists have sculpted 3D printed skulls with clay to reconstruct the faces of more than 900 missing and unidentified homicide victims. In this video published by CNN, artists from around the world work along with the Forensic Anthropology Laboratory to reconstruct faces to identify these victims.
In the film industry, movie labs and companies now are using more widely the technology of 3D printing for makeup preps and special effects to create characters. As an example, artists Steve Yang and Eddie Wang from Alliance Studio are using 3D printing for a new era of special effects and sculpture creation. In this video, they shared their story of how they started to work with 3D printing when everything was using traditional methods and how this technology changed their way of creating things in a way that was not seen before.
Also in this article, Rick Baker the Star Wars famous makeup artist uses 3D printers for the creation of monsters and props. Rick Baker has been able to create parts and scaled copies of his movie characters by using the technology of 3D printing. This technology along with the digital design has helped to decrease the overall time spent for the creation of the movie models.
Many companies around the world are using 3D printing to create exceptional high-precision models for prototyping and industrial manufacturing. 3D offers a less expensive and a very affordable process due to most models being produced using plastic and other variety of materials. Moreover, this innovative method utilizes less material for manufacturing and prototyping compared to traditional techniques.
3D Printing in EducationIn the fields of education, there are countless applications of 3D printing technology with such interesting applications. The past decade has seen explosive growth in STEM education in progressive schools, as theoretical textbook knowledge is being replaced by experiential, project-based learning. When students shaped by this innovative learning ecosystem join the workforce, they are scaling new heights to help transform our manufacturing processes as well. Where appropriate, additive manufacturing technologies like 3D printing are now replacing traditional methods to bring more flexibility, design innovation, and cost savings to production processes.
For instance, in this case Lift 3.0 is using 3D printers in Russia to teach kids the value of additive manufacturing with remarkable results.
Traveling to California, here is the case of John Gardner is a student at Foothill High School in Tustin, CA, who has a great passion for engineering and technology. Once introduced to 3D printers he began to develop his prototypes for an electric skateboard, custom-fit prosthetic limbs, and more. If you are interested in more cases of 3D printing in education, visit here.
Many companies around the world are using 3D printing to create exceptional high-precision models for prototyping and industrial manufacturing. 3D offers a less expensive and a very affordable process due to most models being produced using plastic and other variety of materials. Moreover, this innovative method utilizes less material for manufacturing and prototyping compared to traditional techniques.
To learn more about, why companies are using 3D printing, click here
How to Print with a 3D Printer?
3D printing is changing the way that objects are being produced. To start in the process of 3D printing, you will need to take some steps and considerations. Read on a few below to have an idea of what you need to set up your creation!
The first step is to contemplate your 3D printer options and choose the one that better fits the purpose of your needs. There are a lot of alternatives and manufacturers, you can always compare models, but make sure to choose a printer that has the right features for your projects and plans.
For instance, there are 3D printers that are affordable and rightly designed for education, engineering, and small-batch manufacturing. Make sure your printer has dual extruders that can print simultaneously for a better production capability. This way you can reduce printing time for rapid prototyping. There are particularly good printers that also come with high-resolution cameras, video-assisted calibration systems, and important safety features.
Some other 3D printers are made to build larger industrial originals. These printers are more advanced and have fully enclosed capabilities. Industrial grade 3D printers permit the printing of complex parts and support a variety of filaments and improve even more printing speed. If you need to choose a printer like this, make sure it offers characteristics such as motion controllers, remote user interface, and interchangeable nozzles. If you are looking for a more comprehensive guide on how to choose a 3D printer, visit our 2020 printer buying guide.
Step 2: Choosing a 3D Slicing SoftwareTo create a 3D printed object modeling software is needed. There are a lot of websites and providers that offer free downloadable software programs to design and model, and others that offer a variety of 3D models or mockups that other people have used to create their replicas. Research and look for a slicing software that is intuitive, user-friendly, and has customized advanced features. One important point too is to make sure that the software that you prefer also supports a multi-lingual interface in case you need it.
The next step is to set the design ready for the printer. When the printer receives the data from the software it sends the signal to the printer to start building the item using a filament that is like a cord that passes to the plates of the printer. The most commonly used file format for 3D printing designs is STL, (Standard Triangle Language). The original design when being printed is translated into several triangles in a 3D printing space, which sets up for the printers and related hardware to construct the resulting object. The resolution of a file is recommended to be in an optimal size so the machines and software can work smoothly to create your final product.
In this last process, the object is created through layering. One layer by another is added until the shape and final object is formed. The process of repeatedly printing over the same area is called Fused Depositional Model (FDM). The most common material for 3D printing is plastic, but there are a lot of other materials that can be used and adopted by 3D printers such as PLA, ABS, HIPS, carbon fiber enforced, flexibles, and much more.
Where to Find 3D Printing Files?If you are looking to obtaining files for 3D printing, there are a lot of websites that offer these files, some of them for free. A variety of STL files, 3D printed models, 3D printed files and 3D printing designs in other file formats can be found if you surface the website. Shown below, here is a brief list of some sites that provide files and resources for 3D printing.
- ALL3DP visit site.
- Pinshape visit site.
- MyMiniFactory visit site.
As time progresses, there are more and more uses for 3D printing that shows light of phenomenal events thanks to the usage of 3D printers. Many people believe 3D printing will announce a revolution in the manufacturing industry and the world economy. Although 3D printing has certain limitations, this advanced technology is now universally adopted by big corporations as a crucial mainstay of the manufacturing industry.
Connect with Raise3D:
Have you had a great experience with Raise3D that you would like to share? Please contact us at [email protected]. We look forward to hearing from you.
For more information about Raise3D printers and services, browse our website, or schedule a demo with one of our 3D printing experts.
The Complete Guide to 3D Printing [Part 2]
3D printing is used in a variety of industries, both for rapid prototyping and short-term production.
A key application of 3D printing in various industries is the rapid prototyping of new parts during R&D. No other technology has the capability to instantly produce plastic or metal parts - even in non-factory conditions.
3D printers can be used in-house by companies, while some businesses prefer to order 3D printed prototypes through service bureaus.
Medical
3D printing can be used to make medical components such as titanium implants and surgical guides (SLM), 3D printed prostheses (SLS, FDM) and even 3D bioprinted human tissues. Components for medical equipment and technology - X-ray machines, MRI, etc. - can also be made by 3D printing.
SLA and SLS technologies are also widely used in the dental industry for model making, prostheses and restorations.
Aerospace industry
The aerospace industry has become a major consumer of 3D printing technology because it can produce very light parts with an excellent strength-to-weight ratio. Examples include things as simple as cab bulkheads (SLS) and down to revolutionary engine components (SLM) such as the 3D printed fuel injector tip designed and manufactured by GE.
Cars
Automotive companies regularly use 3D printers to make one-off parts and repairs, as well as rapid prototypes. Common 3D printed automotive parts include brackets, dashboard components, and antennas (FDM).
More extreme examples include vehicles with large 3D printed metal structural components, such as early models from automotive startup Divergent.
Jewelry and art
3D printing technologies such as SLA are widely used (as an indirect fabrication process) in the production and repair of jewelry, while almost all types of 3D printers can be used to create art and sculpture.
Construction
Advances in additive manufacturing with high quality workmanship have expanded the scope of applications in construction and architecture. Concrete 3D printing, which is a bit like FDM but with very wide nozzle extruders, plays an important role in this industry, but more common 3D printing technologies such as SLM can be used for products such as bridge structures.
3D printing file formats:
3D printing parts can be designed with standard CAD software, but 3D printers can only read certain file formats. There are four main file formats for 3D printing.
STL: The most common file format for 3D printers, STL contains part geometry information in the form of tessellated triangles. It does not contain information such as color, material, or texture. The file size is proportional to the detail, which can be a problem.
OBJ: Less common than STL, the OBJ file format encodes the geometry of a 3D model and can include curves and free-form surfaces in addition to tessellation. It can also contain color, material, and texture information, making it useful for full color processes.
3MF: Invented by Microsoft, 3MF is an XML-based format with small file sizes and a good level of error prevention. It has not yet been widely adopted, but is supported by companies such as Stratasys, 3D Systems, Siemens, HP, and GE.
AMF: The successor to the STL format, AMF is much more compact and allows you to tessellate both curved and flat triangles, making it much easier to encode parts of various shapes. Since its inception, the format has been slowly adopted.
3D printing settings and specifications:
3D printing uses specific terminology that may not be clear to beginners. These terms refer to printer settings and/or specifications that can affect how 3D printed parts turn out.
Infill
When making 3D printed parts, it may be necessary to specify an infill percentage, which refers to the internal density of the part. A low infill percentage will result in a mostly hollow part with minimal material holding the mold together; a high infill percentage will result in a stronger, denser, and heavier part.
Layer Height
Layer height, sometimes referred to as z-resolution, is the distance between one 2D part layer and the next. A smaller layer height means finer resolution (and higher possible level of detail) along the z-axis, i.e. top down. A low layer height is an indication of a high quality printer, but users can set a higher layer height for faster, more economical printing.
Print speed
The printer's print speed, measured in millimeters per second, indicates the speed at which the machine can process the source material. Like the layer height, this value can either be a measure of the printer's maximum speed or be user-defined: slower print speeds usually result in more accurate prints.
Print temperature
When applied to processes such as FDM, print temperature usually refers to the temperature of the hot end, the part of the print head that heats the thermoplastic filament. Some FDM printers are also equipped with a heated print bed, the temperature of which is specified by the manufacturer. In both cases, the temperature is usually controlled by the user.
Resolution
In 3D printing, resolution almost always refers to the smallest possible movement along the X and Y axes (width and depth) of either the laser beam (SLA, SLM, etc.) or the print head (FDM). This value is more difficult to measure than the height of the layer, and it is not always proportional to it.
Shells
Like wall thickness in injection molding, shell (or shell thickness) refers to the outer wall thickness of the 3D printed part. When 3D printing, users usually have to choose the number of shells: one shell = outer walls as thick as a 3D printer nozzle; 2 shells = twice the thickness, etc.
Color 3D printing:
Since 3D printing is primarily used as a prototyping tool, single color prints are sufficient for most applications. However, there are several options for color 3D printing, including high-end material inkjet printers, multi-extruder FDM printers, and post-processing options.
Inkjet Printing Technologies
Major 3D printing companies such as Stratasys, 3D Systems and Mimaki have developed 3D inkjet printers for printing materials and binders that can print 3D models in full color as well as 2D inkjet printers. However, these machines are expensive and the parts do not always have excellent mechanical properties.
Multiextrusion
Several FDM 3D printers are equipped with two (or more) printheads, allowing you to simultaneously print on two spools of filament - different colors or even different materials - within the same print job. It's simple and affordable, but usually limited to two colors.
Filament replacement
Single extruder FDM 3D printer can produce multi-color prints. To do this, you need to pause printing at certain points and replace the spool of thread with a thread of a different color. This is a very slow method of applying color and does not allow precise control over where each color goes.
Adding color after printing
Many 3D printed parts can be dyed, tinted or painted after printing. While this adds another step to the process, it often strikes the best balance between quality and economy.
Post-Processing 3D Printed Parts:
Many 3D printed parts require at least some level of post-processing after leaving the print bed. This may include important processes such as the removal of supports, or additional cosmetic processes such as painting. Some processes apply to all or most 3D printing technologies, and some are specific to a particular technology.
Support Removal
3D printing technologies such as FDM and SLA require the installation of support structures (vertical struts between the printed layer and the part itself) to keep the printed object from breaking during the manufacturing process.
These supports must be removed when the part is finished. Some printers, such as dual-extrusion FDM machines, can print dissolvable support structures, allowing the support structures to be easily detached from the part using liquid chemicals. Insoluble supports must be manually cut from the part, leaving a mark that may need to be sanded down.
Washing and removing the powder
Some 3D printing technologies (such as SLA) leave sticky marks on parts, while others (SLM, SLS) may leave powder marks. In these cases, the parts must be washed - manually or with a special machine - or the powder removed with compressed air.
Heat treatment
Many of the key 3D printing technologies print parts from materials that are not yet in their final chemical state after leaving the printing mold. Such details are sometimes called "green".
Many 3D printed metal parts require heat treatment after printing to increase layer fusing and remove contaminants. And bonded inkjet 3D printers, for example, produce parts that need to be stripped and sintered after printing to remove resin bond layers from inside metal parts.
Some 3D printed resin parts require post-curing after printing to increase their hardness and make them usable.
Surface Treatment
3D printed parts can be subjected to a wide range of surface treatments, from textural treatments such as sanding and smoothing, to visual treatments such as painting and toning. Some technologies, such as FDM, can create a rather rough surface that requires sanding, while others, such as SLA, produce a much smoother surface.
Combination of 3D printing with other technologies:
3D printing does not have to be used as a separate process. Rather than being seen as a competitor to CNC machining and injection molding, it can actually complement these and other manufacturing processes.
Combination examples include:
- 3D print the main body of the part and then CNC mill the thin parts to tighter tolerances;
- 3D printable master pattern for investment casting or vacuum casting;
- 3D print the part and then injection mold it using injection molding.
There are hybrid manufacturing systems that combine 3D printing with other technologies. For example, Mazak's INTEGREX i-400 AM and DMG MORI's Lasertec DED can perform both 3D printing and CNC milling.
Will 3D printing replace other manufacturing processes?
Analysts have long speculated about whether 3D printing could replace other manufacturing processes, including:
- Processing;
- Molding;
- Casting.
However, despite the desire of AM equipment manufacturers to position 3D printing as an end-to-end manufacturing technology, in practice, 3D printing is still limited to some specific manufacturing operations, especially low-volume production of specific materials.
In some areas, 3D printing has certainly overtaken other processes. For example, rapid prototyping with inexpensive plastics like ABS now dominates 3D printing, as ABS is cheaper to print than machined. 3D printing also seems to have established itself as the ideal tool for making objects such as patient-specific titanium medical implants: the speed and geometric flexibility of 3D printing is hard to beat in these specific situations.
In addition, 3D printing is an ideal tool for making objects such as patient-specific titanium medical implants.
Despite this, processes such as CNC machining currently remain the best for producing high quality parts and prototypes from engineering materials such as POM, PEI, PPS and PEEK, with surface finishes far superior to 3D printing. . In addition, processes such as injection molding are still infinitely faster for mass production of simple plastic parts.
In addition, although additive manufacturing is one of the most significant technological advances in manufacturing, which allows it to take a stronger position in manufacturing in general, more established processes such as CNC and injection molding are also being improved to produce higher quality parts. .
3D printing will continue to take an increasing share of manufacturing jobs, but it will not completely replace other technologies.
What did 3D printing look like 10 years ago?
A decade ago, the nascent 3D printing industry was gearing up for what it believed would be a 3D printing revolution: a 3D printer in every home, allowing families to print new items they might need, such as a spare part for a refrigerator, a new toy for kids, or even components to build a second 3D printer.
In 2012-2014, FDM 3D printer manufacturers such as MakerBot actively promoted their 3D printers in the consumer market, trying to convince ordinary people that a 3D printer can improve their home life and work. However, it was clear that these companies were trying to exploit the novelty factor of 3D printing and that their products had no practical application; a 2012 MakerBot press release seems to prove it: Make an entire chess set at the touch of a button. Friends, classmates, colleagues and family members will see what you are doing and say "Wow!".
Just a few years later, this so-called 3D printing revolution clearly failed, and many 3D printer manufacturers began to rethink their goals, moving from consumer to professional and industrial markets, where there were more concrete (and profitable) applications of additive technology.
In addition, those who were already working in the professional and industrial fields - companies such as 3D Systems and Stratasys - began to try to destroy the idea of 3D printing as a prototyping technology, positioning it as a viable mass production tool (which, obviously, , could be more profitable for the 3D printing industry, as manufacturers would have to fill entire factories with 3D printers, buy 3D printer management software, and hire 3D printing consultants).
What will 3D printing look like in 10 years?
3D printing companies have abandoned the prospect of putting a 3D printer in every home. However, in 10 years, they can expect some form of additive manufacturing to appear in more factories.
Although there is less talk about 3D printing today than in 2012, the technology continues to gain momentum in the professional and industrial world.
According to a recent report, market research firm 3DPBM Research expects the value of additive metal manufacturing to rise from $1.6 billion in 2020 to $30 billion by 2030, and this is largely due to the repositioning of AM as a manufacturing tool and the development of more high-performance engineering materials. (That said, 3D printing will remain a valuable prototyping tool in many industries, and prototyping applications will benefit just as much from technological advances.)
However, not only metal AM is being developed. Technologies such as HP's Multi Jet Fusion have opened up new possibilities for plastic printing, and innovators such as Carbon have developed new high-speed processes in the photopolymerization category. Niche areas such as 3D bioprinting and micro 3D printing are also regularly opening up new opportunities, and composite 3D printing (such as continuous carbon fiber 3D printing) is also on the rise: IDTechEX predicts that by 2030, the market size of composite 3D printing will be $1.7 billion
In short, 3D printing will gradually become a serious competitor to other manufacturing processes in many disciplines.
How to outsource 3D printing services?
Investments in 3D printing hardware and software are not suitable for all businesses, so many successful companies outsource their 3D printing needs to third parties, such as online 3D printing service bureaus (for one-time projects) or prototyping partners and production, such as 3ERP (for one-time projects or repeat orders).
When outsourcing 3D printing services, it is important to consider whether your business needs design and manufacturing services or just manufacturing services. (Keep in mind that a poorly executed 3D model may fail for 3D printing).
In general, though, ordering 3D printed parts from a third party is easier than ever. Many manufacturers can start 3D printing with just a digital 3D model, although more important projects may require a technical drawing to communicate additional information such as materials, colors, and tolerances. Some 3D printing service providers (including 3ERP) will offer advice on suitable 3D printing technologies and materials for your project.
Explore our full range of 3D printing services, including available technologies and materials, or request a quote for your 3D printing project.
3D printing for dummies or "what is a 3D printer?"
- 1 3D printing term
- 2 3D printing methods
- 2.1 Extrusion printing
- 2.2 Melting, sintering or gluing
- 2.3 Stereolithography
- 2.4 Lamination
- 3 Fused Deposition Printing (FDM)
- 3.
1 Consumables
- 3.2 Extruder
- 3.3 Working platform
- 3.4 Positioners
- 3.5 Control
- 3.6 Varieties of FDM printers
- 4 Laser stereolithography (SLA)
- 4.1 Lasers and projectors
- 4.2 Cuvette and resin
- 4.3 Varieties of Stereolithography Printers
The term 3D printing
The term 3D printing has several synonyms, one of which quite briefly and accurately characterizes the essence of the process - "additive manufacturing", that is, production by adding material. The term was not coined by chance, because this is the main difference between multiple 3D printing technologies and the usual methods of industrial production, which in turn received the name "subtractive technologies", that is, "subtractive". If during milling, grinding, cutting and other similar procedures, excess material is removed from the workpiece, then in the case of additive manufacturing, material is gradually added until a solid model is obtained.
Soon 3D printing will even be tested on the International Space Station
Strictly speaking, many traditional methods could be classified as "additive" in the broad sense of the word - for example, casting or riveting. However, it should be borne in mind that in these cases, either the consumption of materials is required for the manufacture of specific tools used in the production of specific parts (as in the case of casting), or the whole process is reduced to joining ready-made parts (welding, riveting, etc.). In order for the technology to be classified as “3D printing”, the final product must be built from raw materials, not blanks, and the formation of objects must be arbitrary - that is, without the use of forms. The latter means that additive manufacturing requires a software component. Roughly speaking, additive manufacturing requires computer control so that the shape of final products can be determined by building digital models. It was this factor that delayed the widespread adoption of 3D printing until the moment when numerical control and 3D design became widely available and highly productive.
3D printing methods
3D printing technologies are numerous, and there are even more names for them due to patent restrictions. However, you can try to divide technologies into main areas:
Extrusion printing
This includes methods such as deposition fusion (FDM) and multi-jet printing (MJM). This method is based on the extrusion (extrusion) of consumables with the sequential formation of the finished product. As a rule, consumables consist of thermoplastics or composite materials based on them.
Melting, sintering or bonding
This approach is based on bonding powdered material together. Formation is done in different ways. The simplest is gluing, as is the case with 3D inkjet printing (3DP). Such printers deposit thin layers of powder onto the build platform, which are then selectively bonded with a binder. Powders can be made up of virtually any material that can be ground to a powder—plastic, wood, metal.
This model of James Bond's Aston Martin was successfully printed on a Voxeljet SLS printer and blown up just as successfully during the filming of Skyfall instead of the expensive original
sintering (SLS and DMLS) and smelting (SLM), which allow you to create all-metal parts. As with 3D inkjet printing, these devices apply thin layers of powder, but the material is not glued together, but sintered or melted using a laser. Laser sintering (SLS) is used to work with both plastic and metal powders, although metal pellets usually have a more fusible shell, and after printing they are additionally sintered in special ovens. DMLS is a variant of SLS installations with more powerful lasers that allow sintering metal powders directly without additives. SLM printers provide not just sintering of particles, but their complete melting, which allows you to create monolithic models that do not suffer from the relative fragility caused by the porosity of the structure. As a rule, printers for working with metal powders are equipped with vacuum working chambers, or they replace air with inert gases. Such a complication of the design is caused by the need to work with metals and alloys subject to oxidation - for example, with titanium.
Stereolithography
How an SLA printer works
Stereolithography printers use special liquid materials called "photopolymer resins". The term "photopolymerization" refers to the ability of a material to harden when exposed to light. As a rule, such materials react to ultraviolet irradiation.
Resin is poured into a special container with a movable platform, which is installed in a position near the surface of the liquid. The layer of resin covering the platform corresponds to one layer of the digital model. Then a thin layer of resin is processed by a laser beam, hardening at the points of contact. At the end of illumination, the platform together with the finished layer is immersed to the thickness of the next layer, and illumination is performed again.
Lamination
Laminating (LOM) 3D printers workflow
Some 3D printers build models using sheet materials - paper, foil, plastic film.
Layers of material are glued on top of each other and cut along the contours of the digital model using a laser or a blade.
These machines are well suited for prototyping and can use very cheap consumables, including regular office paper. However, the complexity and noise of these printers, coupled with the limitations of the models they produce, limit their popularity.
Fused deposition modeling (FDM) and laser stereolithography (SLA) have become the most popular 3D printing methods used in the home and office.
Let's take a closer look at these technologies.
Fused Deposition Printing (FDM)
FDM is perhaps the simplest and most affordable 3D construction method, which makes it very popular.
High demand for FDM printers is driving device and consumable prices down rapidly, along with technology advances towards ease of use and improved reliability.
Consumables
ABS filament spool and finished model
FDM printers are designed to print with thermoplastics, which are usually supplied as thin filaments wound on spools. The range of "clean" plastics is very wide. One of the most popular materials is polylactide or "PLA plastic". This material is made from corn or sugar cane, which makes it non-toxic and environmentally friendly, but makes it relatively short-lived. ABS plastic, on the other hand, is very durable and wear-resistant, although it is susceptible to direct sunlight and can release small amounts of harmful fumes when heated. Many plastic items that we use on a daily basis are made from this material: housings for household appliances, plumbing fixtures, plastic cards, toys, etc.
In addition to PLA and ABS, printing is possible with nylon, polycarbonate, polyethylene and many other thermoplastics that are widely used in modern industry. More exotic materials are also possible, such as polyvinyl alcohol, known as "PVA plastic". This material dissolves in water, which makes it very useful for printing complex geometric patterns. But more on that below.
Model made from Laywoo-D3. Changing the extrusion temperature allows you to achieve different shades and simulate annual rings
It is not necessary to print with homogeneous plastics. It is also possible to use composite materials imitating wood, metals, stone. Such materials use all the same thermoplastics, but with impurities of non-plastic materials.
So, Laywoo-D3 consists partly of natural wood dust, which allows you to print "wooden" products, including furniture.
The material called BronzeFill is filled with real bronze, and models made from it can be ground and polished, achieving a high similarity to products made from pure bronze.
One has only to remember that thermoplastics serve as a binding element in composite materials - they determine the thresholds of strength, thermal stability and other physical and chemical properties of finished models.
Extruder
Extruder - FDM print head. Strictly speaking, this is not entirely true, because the head consists of several parts, of which only the feed mechanism is directly "extruder". However, by tradition, the term "extruder" is commonly used as a synonym for the entire print assembly.
FDM extruder general design
The extruder is designed for melting and applying thermoplastic thread. The first component is the thread feed mechanism, which consists of rollers and gears driven by an electric motor. The mechanism feeds the thread into a special heated metal tube with a small diameter nozzle, called a "hot end" or simply a "nozzle". The same mechanism is used to remove the thread if a change of material is needed.
The hot end is used to heat and melt the thread fed by the puller. As a rule, nozzles are made from brass or aluminum, although more heat-resistant, but also more expensive materials can be used. For printing with the most popular plastics, a brass nozzle is quite enough. The “nozzle” itself is attached to the end of the tube with a threaded connection and can be replaced with a new one in case of wear or if a change in diameter is necessary. The nozzle diameter determines the thickness of the molten filament and, as a result, affects the print resolution. The heating of the hot end is controlled by a thermistor. Temperature control is very important, because when the material is overheated, pyrolysis can occur, that is, the decomposition of plastic, which contributes both to the loss of the properties of the material itself and to clogging of the nozzle.
PrintBox3D One FDM Printer Extruder
In order to prevent the filament from melting too early, the top of the hot end is cooled by heatsinks and fans. This point is of great importance, since thermoplastics that pass the glass transition temperature significantly expand in volume and increase the friction of the material with the walls of the hot end. If the length of such a section is too long, the pulling mechanism may not have enough strength to push the thread.
The number of extruders may vary depending on the purpose of the 3D printer. The simplest options use a single printhead. The dual extruder greatly expands the capabilities of the device, allowing you to print one model in two different colors, as well as using different materials. The last point is important when building complex models with overhanging structural elements: FDM printers cannot print “over the air”, since the applied layers require support. In the case of hinged elements, temporary support structures have to be printed, which are removed after printing is completed. The removal process is fraught with damage to the model itself and requires accuracy. In addition, if the model has a complex structure with internal cavities that are difficult to access, building conventional supports may not be practical due to the difficulty in removing excess material.
Finished model with PVA supports (white) before and after washing
In such cases, the same water-soluble polyvinyl alcohol (PVA) comes in handy. Using a dual extruder, you can build a model from waterproof thermoplastic using PVA to create supports.
After printing, PVA can simply be dissolved in water and a complex product of perfect quality can be obtained.
Some FDM printers can use three or even four extruders.
Work platform
Heated platform covered with removable glass work table
Models are built on a special platform, often equipped with heating elements. Preheating is required for a wide range of plastics, including the popular ABS, which are subject to a high degree of shrinkage when cooled. The rapid loss of volume by cold coats compared to freshly applied material can lead to model distortion or delamination. The heating of the platform makes it possible to significantly equalize the temperature gradient between the upper and lower layers.
Heating is not recommended for some materials. A typical example is PLA plastic, which requires a fairly long time to harden. Heating PLA can lead to deformation of the lower layers under the weight of the upper ones. When working with PLA, measures are usually taken not to heat up, but to cool the model. Such printers have characteristic open cases and additional fans blowing fresh layers of the model.
Calibration screw for work platform covered with blue masking tape
The platform needs to be calibrated before printing to ensure that the nozzle does not hit the applied layers and move too far causing air-to-air printing resulting in plastic vermicelli. The calibration process can be either manual or automatic. In manual mode, calibration is performed by positioning the nozzle at different points on the platform and adjusting the platform inclination using the support screws to achieve the optimal distance between the surface and the nozzle.
As a rule, platforms are equipped with an additional element - a removable table. This design simplifies the cleaning of the working surface and facilitates the removal of the finished model. Stages are made from various materials, including aluminum, acrylic, glass, etc. The choice of material for the manufacture of the stage depends on the presence of heating and consumables for which the printer is optimized.
For a better adhesion of the first layer of the model to the surface of the table, additional tools are often used, including polyimide film, glue and even hairspray! But the most popular tool is inexpensive, but effective masking tape. Some manufacturers make perforated tables that hold the model well but are difficult to clean. In general, the expediency of applying additional funds to the table depends on the consumable material and the material of the table itself.
Positioning mechanisms
Scheme of operation of positioning mechanisms
Of course, the print head must move relative to the working platform, and unlike conventional office printers, positioning must be carried out not in two, but in three planes, including height adjustment.
Positioning pattern may vary. The simplest and most common option involves mounting the print head on perpendicular guides driven by stepper motors and providing positioning along the X and Y axes.
Vertical positioning is carried out by moving the working platform.
On the other hand, it is possible to move the extruder in one plane and the platforms in two.
SeemeCNC ORION Delta Printer
One option that is gaining popularity is the delta coordinate system.
Such devices are called "delta robots" in the industry.
In delta printers, the print head is suspended on three manipulators, each of which moves along a vertical rail.
The synchronous symmetrical movement of the manipulators allows you to change the height of the extruder above the platform, and the asymmetric movement causes the head to move in the horizontal plane.
A variant of this system is the reverse delta design, where the extruder is fixed to the ceiling of the working chamber, and the platform moves on three support arms.
Delta printers have a cylindrical build area, and their design makes it easy to increase the height of the working area with minimal design changes by lengthening the rails.
In the end, everything depends on the decision of the designers, but the fundamental principle does not change.
Control
Typical Arduino-based controller with add-on modules
FDM printer operation, including nozzle and platform temperature, filament feed rate, and stepper motors for positioning the extruder, is controlled by fairly simple electronic controllers. Most controllers are based on the Arduino platform, which has an open architecture.
The programming language used by printers is called G-code (G-Code) and consists of a list of commands executed in turn by the 3D printer systems. G-code is compiled by programs called "slicers" - standard 3D printer software that combines some of the features of graphics editors with the ability to set print options through a graphical interface. The choice of slicer depends on the printer model. RepRap printers use open source slicers such as Skeinforge, Replicator G and Repetier-Host. Some companies make printers that require proprietary software.
Program code for printing is generated using slicers
As an example, we can mention Cube printers from 3D Systems. There are companies that offer proprietary software but allow third-party software, as is the case with the latest generation of MakerBot 3D printers.
Slicers are not designed for 3D design per se. This task is done with CAD editors and requires some 3D design skills. Although beginners should not despair: digital models of a wide variety of designs are offered on many sites, often even for free. Finally, some companies and individuals offer 3D design services for custom printing.
Finally, 3D printers can be used in conjunction with 3D scanners to automate the process of digitizing objects. Many of these devices are designed specifically to work with 3D printers. Notable examples include the 3D Systems Sense handheld scanner and the MakerBot Digitizer handheld desktop scanner.
MakerBot Replicator 5th Generation FDM Printer with built-in control module on the top of the frame
The user interface of a 3D printer can consist of a simple USB port for connecting to a personal computer. In such cases, the device is actually controlled by the slicer.
The disadvantage of this simplification is a rather high probability of printing failure when the computer freezes or slows down.
A more advanced option includes an internal memory or memory card interface to make the process standalone.
These models are equipped with control modules that allow you to adjust many print parameters (such as print speed or extrusion temperature). The module may include a small LCD display or even a mini-tablet.
Varieties of FDM printers
Stratasys Fortus 360mc professional FDM printer that allows printing with nylon
FDM printers are very, very diverse, ranging from the simplest homemade RepRap printers to industrial installations capable of printing large-sized objects.
Stratasys, founded by FDM inventor Scott Crump, is a leader in industrial plant manufacturing.
You can build the simplest FDM printers yourself. Such devices are called RepRap, where "Rep" indicates the possibility of "replication", that is, self-reproduction.
RepRap printers can be used to print custom built plastic parts.
Controller, rails, belts, motors and other components can be easily purchased separately.
Of course, assembling such a device on your own requires serious technical and even engineering skills.
Some manufacturers make it easy by selling DIY kits, but these kits still require a good understanding of the technology. RepRap Printers
And, despite their "homemade nature", RepRap printers are quite capable of producing models with quality at the level of expensive branded counterparts.
Ordinary users who do not want to delve into the intricacies of the process, but require only a convenient device for household use, can purchase a ready-made FDM printer.
Many companies are focusing on the development of the consumer market segment, offering 3D printers for sale that are ready to print “straight out of the box” and do not require serious computer skills.
3D Systems Cube consumer 3D printer
The most famous example of a consumer 3D printer is the 3D Systems Cube.
While it doesn't boast a huge build area, ultra-fast print speed, or superb model build quality, it's easy to use, affordable, and safe: This printer has received the necessary certification to be used even by children.
Mankati FDM printer demonstration: http://youtu.be/51rypJIK4y0
Laser Stereolithography (SLA)
Stereolithographic 3D printers are widely used in dental prosthetics
Stereolithographic printers are the second most popular and widespread after FDM printers.
These units deliver exceptional print quality.
The resolution of some SLA printers is measured in a matter of microns - it is not surprising that these devices quickly won the love of jewelers and dentists.
The software side of laser stereolithography is almost identical to FDM printing, so we will not repeat ourselves and will only touch on the distinctive features of the technology.
Lasers and projectors
Projector illumination of a photopolymer model using Kudo3D Titan DLP printer as an example
The cost of stereolithographic printers is rapidly declining, due to growing competition due to high demand and the use of new technologies that reduce the cost of construction.
Although the technology is collectively referred to as "laser" stereolithography, most recent developments use UV LED projectors for the most part.
Projectors are cheaper and more reliable than lasers, do not require the use of delicate mirrors to deflect the laser beam, and have higher performance. The latter is explained by the fact that the contour of the whole layer is illuminated as a whole, and not sequentially, point by point, as is the case with laser options. This variant of the technology is called projection stereolithography, "DLP-SLA" or simply "DLP". However, both options are currently common - both laser and projector versions.
Cuvette and resin
Photopolymer resin is poured into a cuvette
A photopolymer resin that looks like epoxy is used as consumables for stereolithography printers. Resins can have a variety of characteristics, but they all share one key feature for 3D printing applications: these materials harden when exposed to ultraviolet light. Hence, in fact, the name "photopolymer".
When polymerized, resins can have a wide variety of physical characteristics. Some resins are like rubber, others are hard plastics like ABS. You can choose different colors and degrees of transparency. The main disadvantage of resins and SLA printing in general is the cost of consumables, which significantly exceeds the cost of thermoplastics.
On the other hand, stereolithographic printers are mainly used by jewelers and dentists who do not need to build large parts but appreciate the savings from fast and accurate prototyping. Thus, SLA printers and consumables pay for themselves very quickly.
An example of a model printed on a laser stereolithographic 3D printer
Resin is poured into a cuvette, which can be equipped with a lowering platform. In this case, the printer uses a leveling device to flatten the thin layer of resin covering the platform just prior to irradiation. As the model is being made, the platform, together with the finished layers, is “embedded” in the resin. Upon completion of printing, the model is removed from the cuvette, treated with a special solution to remove liquid resin residues and placed in an ultraviolet oven, where the final illumination of the model is performed.
Some SLA and DLP printers work in an "inverted" scheme: the model is not immersed in the consumable, but "pulled" out of it, while the laser or projector is placed under the cuvette, and not above it. This approach eliminates the need to level the surface after each exposure, but requires the use of a cuvette made of a material transparent to ultraviolet light, such as quartz glass.
The accuracy of stereolithographic printers is extremely high. For comparison, the standard for vertical resolution for FDM printers is considered to be 100 microns, and some variants of SLA printers allow you to apply layers as thin as 15 microns. But this is not the limit. The problem, rather, is not so much in the accuracy of lasers, but in the speed of the process: the higher the resolution, the lower the print speed. The use of digital projectors allows you to significantly speed up the process, because each layer is illuminated entirely. As a result, some DLP printer manufacturers claim to be able to print with a vertical resolution of one micron!
Video from CES 2013 showing Formlabs Form1 stereolithography 3D printer in action: http://youtu.be/IjaUasw64VE
Stereolithography Printer Options
Formlabs Form1 Desktop Stereolithography Printer
As with FDM printers, SLA printers come in a wide range in terms of size, features and cost.