3D printed microfluidic devices
3D printed microfluidic devices: enablers and barriers
Sidra Waheed,ab Joan M. Cabot,ab Niall P. Macdonald,ab Trevor Lewis,b Rosanne M. Guijt,c Brett Paullab and Michael C. Breadmore*ab
Author affiliations
* Corresponding authors
a
Australian Centre for Research on Separation Sciences (ACROSS), School of Physical Sciences, University of Tasmania, Hobart, TAS, Australia
E-mail:
mcb@utas. edu.au
Fax: +61 3 6226 2858
Tel: +61 3 6226 2154
b ARC Centre of Excellence for Electromaterials Science (ACES), School of Physical Sciences, University of Tasmania, Hobart, TAS, Australia
c Australian Centre for Research on Separation Sciences (ACROSS), Pharmacy School of Medicine, University of Tasmania, Hobart, TAS, Australia
Abstract
3D printing has the potential to significantly change the field of microfluidics. The ability to fabricate a complete microfluidic device in a single step from a computer model has obvious attractions, but it is the ability to create truly three dimensional structures that will provide new microfluidic capability that is challenging, if not impossible to make with existing approaches. This critical review covers the current state of 3D printing for microfluidics, focusing on the four most frequently used printing approaches: inkjet (i3DP), stereolithography (SLA), two photon polymerisation (2PP) and extrusion printing (focusing on fused deposition modeling). It discusses current achievements and limitations, and opportunities for advancement to reach 3D printing's full potential.
- This article is part of the themed collections: Lab on a Chip Recent Review Articles, RACI100: Celebrating Australian Chemistry, 3D Printing and Lab on a Chip 2016 Most Downloaded Articles
Researchers open up low-cost open-source microfluidics 3D printing
0Shares
A team of researchers from the University of Bristol has developed a novel low-cost and open-source 3D printing process for producing microfluidic devices.
A microfluidic chip is a set of micro-channels etched or molded into a material, such as glass, silicon, or in this case, PolyDimethylSiloxane (PDMS), which are connected to the outside world by inputs and outputs pierced through the chip. Through these holes, liquids or gases can be injected and removed by external active or passive systems for biomedical field applications such as laboratories-on-a-chip (LOC), cell biology research, and protein crystallization.
Requiring only simple domestic equipment and a standard desktop 3D printer, and having been developed in free-to-use software, the researchers’ process reduces the cost and complexity of fabricating microfluidics to make the field more accessible.
The team believes their approach could drastically lower the threshold for research and education into microfluidics while making the rapid prototyping of affordable LOC diagnostic technology possible at the point-of-care (POC).
Proposed microfluidic master mould fabrication process.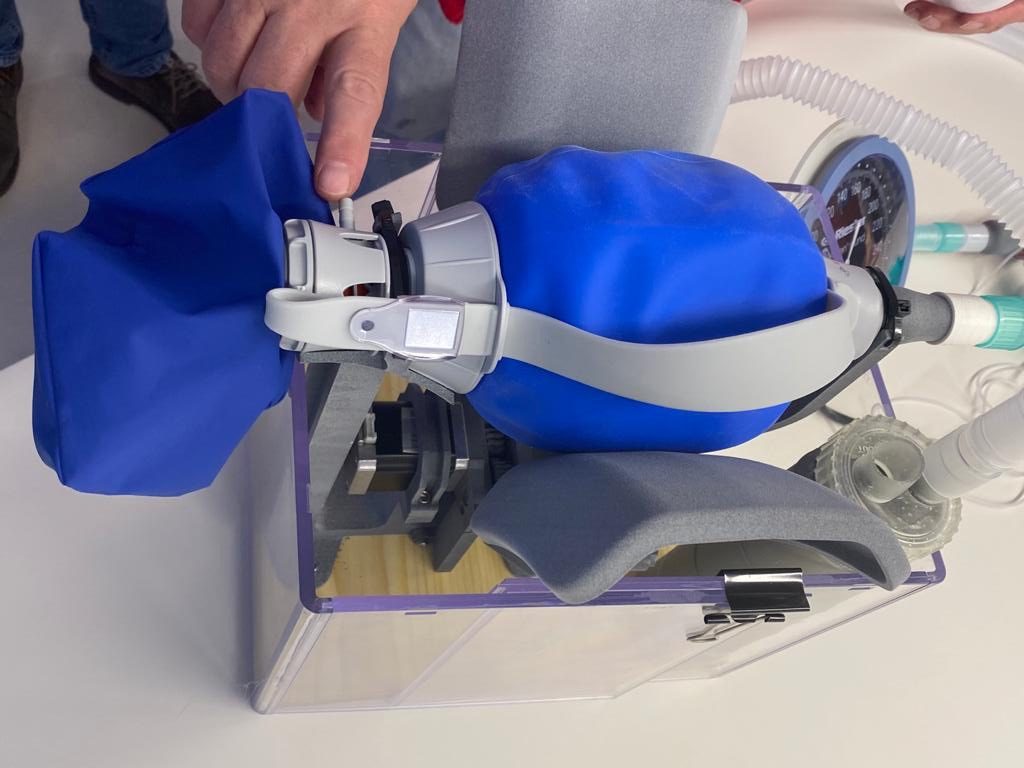
3D printing and microfluidics
For decades, LOC technology has been heralded to answer a range of biological, chemical, and healthcare challenges. However, it is yet to see meaningful adoption and deployment due to the cost both at the research level and the mass-manufacturing stage.
LOC technologies are supported by the field of microfluidics, which is seeing an increasing exploration of 3D printing techniques to advance the technology and increase its accessibility.
In 2018, researchers from New York Genome Center and New York University developed an open-source 3D printed droplet microfluidic control instrument which was reportedly up to 200 times cheaper than other comparable instruments. Designed to identify and target the correct cells to treat diseases such as Rheumatoid Arthritis, the instrument could be obtained and assembled for around $600.
Elsewhere, a process has been developed to 3D print microfluidic devices integrated with fluid handling and functional components. Developed by the Singapore University of Technology and Design, the technique aimed to enable the rapid prototyping of microfluidics for LOC applications in chemical testing and cell analysis.
Most recently, researchers from UC Davis unveiled a new approach to 3D printing using microfluidics, which involved deploying a droplet-based microfluidic system to efficiently 3D print finely-tuned flexible materials. Possible applications for the technology include soft robotics, tissue engineering, and wearable technology.
Material printed with UC Davis’ new droplet-based 3D printing method. Photo via Jiandi Wan.Fabricating the microfluidic devices
The researchers began by 3D printing interconnecting microchannel scaffolds with an Ultimaker 3 Extended 3D printer using PLA, which were then thermally bonded to a glass substrate in the desired configuration to create a microfluidic device master mold.
The microchannels were designed in a range of modular patterns, with each featuring interlocking ball-socket connector ends, using Ultimaker’s Cura open-source slicing software. These ends were developed to mimic puzzle pieces. Successive modules could be arranged in any desired configuration, enabling creating more sophisticated microfluidic systems using a small number of simple modules. A key aspect of this part of the researchers’ process is that it is easy and clear to replicate for non-expert users.
The 3D printed microchannel modules were then mounted onto standard 1mm-thick glass microscope slides into the desired configuration, using the ball-and-socket connectors. The channels were then heated for around a minute to bind them to the glass with a weighted slide placed on top to prevent deforming and shrinking. After heating, the slides were partially fused and placed weighted-side down onto a metal plate to rapidly cool the weighted slide and remove it from the mold.
The master mold can be used again and again to produce microfluidic devices in PDMS. Post-printing, the master mold fabrication process can be completed in less than five minutes, allowing the method to be used for both formal and informal learning environments.
Open-source microfluidics
To ensure the proposed technique is fully democratized, the researchers developed an open-source Autodesk Fusion add-in which allows any user to design and export interconnecting microfluidic channel scaffolds for 3D printing. Using this plugin, a user can go from a microfluidic channel design to a completed microfluidic channel without requiring CAD software expertise or time and resource-intensive techniques or equipment.
Users can prototype interconnecting microfluidic channels down to 100 μm resolution in width, either through printing their own designs or choosing from a vast library of microchannel scaffolds listed in the add-in. A protocols.io instruction set has also been made available detailing the researchers’ full process, with links to the up-to-date add-in and profiles.
Using this technique, users can fabricate microfluidic devices from PDMS with only household equipment and no hazardous chemicals, thereby making microfluidic experimentation accessible to schools, hobbyists, and researchers no matter their resources. The team hopes that this approach will be adopted by researchers and educators worldwide to “help to inspire the next generation of lab-on-a-chip developers.”
Furthermore, the researchers believe their technique could pave the way for “truly affordable” LOC healthcare diagnostic testing, which can be performed at the point-of-care, through applying the microfluidic PDMS channels directly to any cleaned glass surface, such as a mobile phone screen or a car windshield.
Further details on the study can be found in the paper titled “Negligible-cost microfluidic device fabrication using 3D printed interconnecting channel scaffolds” published in the Plos One journal. The study is co-authored by H. Felton, R. Hughes, and A. Diaz-Gaxiola.
Subscribe to the 3D Printing Industry newsletter for the latest news in additive manufacturing. You can also stay connected by following us on Twitter and liking us on Facebook.
Looking for a career in additive manufacturing? Visit 3D Printing Jobs for a selection of roles in the industry.
Featured image shows proposed microfluidic master mould fabrication process. Image via University of Bristol.
Tags A. Diaz-Gaxiola H. Felton lab-on-a-chip Microfluidics New York Genome Center New York University R. Hughes Singapore University of Technology and Design UC Davis Ultimaker Ultimaker 3 Ultimaker Cura University of Bristol
Hayley Everett
Hayley is a Technology Journalist for 3DPI and has a background in B2B publications spanning manufacturing, tools and cycling. Writing news and features, she holds a keen interest in emerging technologies which are impacting the world we live in.
overview of the latest advances in medical 3D printing
3D printing has huge potential in medicine. With these technologies, it is possible to reproduce highly accurate 3D models of human organs, as well as some implants. Developers of such technologies strive to create organs in real time. At the end of August 2018, a team from the University of Minnesota announced what is claimed to be the world's first 3D-printed bionic eye. It gives great hope to patients who have lost their sight. At the end of July 2018, smartARM, which developed a self-learning robotic arm prosthesis, won the international technology competition Microsoft Imagine Cup 2018. In May 2018, it became known about the creation of a 3D printer that prints tissue from sugar for growing organs and studying tumors.
3D sugar printer will print organs There are already 3D printers on the market that can print objects out of sugar. In contrast to these devices, the new equipment uses isomalt, a sugar substitute derived from beets and commonly found in lozenges for sore throats and coughs.
After dissolving and 3D printing, the sugar structures cool and solidify, creating a strong scaffold - carrier substrates, on the basis of which living cells are cultivated. Here one of the problems is getting material that will “leave” not earlier and not later than the right time.
Sugar 3D printing gets tricky when it comes to regenerating heart tissue. Too much pressure causes the structure to lose its shape, and too much heat causes the tissue to crystallize or burn. Isomalt is less prone to crystallization than regular sugar and does not discolor when dissolved.
Professor Rohit Bhargava, who works at the Cancer Center in Illinois, says the unique method makes it possible to produce structures from thin tubes with a round cross section. Previously, this was not possible for polymers. Soluble sugar, on the other hand, helps create cylinders and tunnels that resemble blood vessels. It is through these vessels that nutrients can be transported to tissues or cells. The development of a new method will also allow the creation of channels in microfluidic devices.
University of Illinois technology can be used in areas such as medical research, biomedical engineering, and manufacturing. Experts hope that over time their 3D printer will be able to print human organs from scratch.
First 3D printed cornea
In May 2018, the first 3D printed cornea was announced. This achievement was able to boast at the University of Newcastle (Newcastle University). They can now use 3D printing to form stromal cell corneas for each individual, the researchers say.
According to the Financial Times, Newcastle University researchers have created a special bio-ink consisting of corneal stroma cells from a living donor, alginate (polysaccharide) and collagen, a protein that forms the basis of the body's connective tissue. By loading this substance into a conventional 3D printer, it was possible to print a healthy cornea in just 10 minutes. Moreover, after printing, more than 90% of the cells remained viable, and on the seventh day - 83%.
British scientists create the first 3D printed corneas for the human eye
The composition of the ink must be flexible, yet rigid enough for the finished cornea to retain its shape.![]() |
This most convex transparent part of the eyeball can suffer from infections, burns, mechanical trauma and other causes. By May 2018, about 10 million people around the world are in need of a corneal transplant to avoid losing their sight, and another 5 million people are already blind, but have a chance to recover from such a transplant. Unfortunately, donor material is always in short supply, but the development of Newcastle University scientists can solve this problem. True, the technology still has to undergo clinical trials, and it may take about 5 years before mass use, the researchers believe.
Handheld 3D printer to print artificial skin directly on a person
In May 2018, researchers at the University of Toronto introduced a handheld 3D skin printer designed to treat deep burn wounds. The research team notes that this is the first device that forms and positions a printed tissue sample directly at the burn site in just a couple of minutes. Read more here.
AI 3D organ printing software
On April 11, 2018, biological 3D printer manufacturer Aether announced the release of artificial intelligence (AI)-based medical imaging software that will significantly advance the development of 3D organ printing. The new Automatic Segmentation and Reconstruction (ASAR) will help clinicians and researchers increase productivity through automated segmentation of organs and tissues.
Hospitals save tens of thousands of dollars with 3D printing
In March 2018, the North Manchester Hospital (NMGH) opened a 3D printing lab to assist oral and maxillofacial surgeons in the treatment and rehabilitation of patients with head and neck cancer, facial trauma or congenital anomalies. The lab was created by reconstructive technologist Oliver Burley, who made a case for the economic benefits of a 3D lab for the hospital, as well as a fundraiser for the lab itself, software, and a $42,000 PolyJet 3D printer. The laboratory currently employs three specialists who work with nine maxillofacial surgeon consultants.
After earning a Master's degree in Reconstructive Technology, in which he studied the application of 3D printing, Burleigh presented a 3D business model to Manchester Hospital management. The first argument in her favor was cost savings, as the hospital spent $166,000 annually on 3D printing projects. On average, a hospital encounters 20 cancer cases and 8-10 injuries each year, and analysis based on these data showed that it would be cheaper to run an in-house 3D lab. Although the hospital has to pay for laboratory licensing, this amount remains fixed and does not depend on the amount of work done. The second argument was the saving of time for surgeons, who can use 3D models when planning operations. Finally, the last argument was the reduction in the delivery time of 3D models from the manufacturer.
3D printing technology appeared thanks to the discovery of the American inventor Charles Hull in 1983 and gradually spread to all areas of production.
Materialize Mimics Innovation Suite was selected as modeling software and ProPlan CMF was used to create skull bone reconstruction models for mandibular osteotomies; the software cost about $25,000. Burley notes that 3D models are used by the hospital in almost every case of head and neck cancer; he is confident that in five years, the 3D printing laboratory will become a must-have application for treatment and rehabilitation centers for cancer patients.
The laboratory primarily works with patients with head or neck cancer who require reconstructive surgeries, including those based on bone grafts for the reconstruction of the upper or lower jaw. The patient's head is scanned and then a virtual 3D model is created. Surgeons and laboratory specialists can review various types of reconstructive surgeries and devices in virtual reality before moving on to the design stage. Designed prostheses, rods or plates are 3D printed using metal or plastic mixtures. The final stage of the project assumes that the resulting model is sterilized and transferred to surgeons. Thanks to a wide range of instruments, the laboratory also accepts other orders and is already used by orthopedists, neurologists and rheumatologists.
The UK NHS has made significant strides in 3D printing, with Wales recently creating the world's first combined bone graft and presenting a 3D model of the operation. Experts note the prerequisites that three-dimensional printing will be increasingly used in healthcare. In February 2018, a new research center Bristol Biomedical Research Center (BRC) opened in Bristol, which is supposed to study tissue engineering technologies through bioprinting. New developments will be based on data from existing studies on the cardiovascular system and 3D printed cardiac implants.
3D printed limbs being inserted into Syrian refugees
In March 2018, it was reported that a Jordanian hospital was using 3D printed limbs to treat refugees wounded in Syria. The technologies used in the project of the international medical organization Doctors Without Borders (Medecins Sans Frontieres, MSF) make it possible to design and manufacture a prosthesis within 24 hours, and its cost is several times lower than that of traditional artificial limbs.
Since the beginning of 2017, five volunteer patients, including children, have participated in the MSF program, according to New Atlas. The project is being implemented at the MSF Reconstructive Surgery Center at Al-Mowasah Hospital in the Jordanian capital Amman, where the wounded during the military conflicts in Syria, Iraq and Yemen are being treated.
Jordan hospital uses 3D printed limbs to treat refugees wounded in Syria
We do not use overly complex electronic solutions, but strive to make as simple as possible, but at the same time reliable prostheses, said Safa Herfat, MSF engineer for biomedical equipment, in an interview with the publication. |
According to him, a 3D printed arm can cost about $20, while a conventional prosthetic upper limb costs hundreds of dollars.
In addition to the low cost and speed of production, there are other advantages. For example, a 3D-printed prosthesis can be designed to fit the individual needs of the patient and their daily tasks, from driving a car to ordinary household chores. In addition, the design can be carried out remotely, and only the limb scanning and 3D printing itself can be performed on site.
Printed prostheses are also significantly lighter than traditional ones, which is important for patients.
If the prosthesis is heavy and bulky, the patient, most likely, does not pass with it for a long time and will stop using it, - said Safa Herfat. |
MSF hopes to expand the program to other areas that need similar assistance.
Diabetes 3D Bioprinter
In early December 2017, the Australian University of Wollongong introduced a new customizable 3D bioprinter that could improve the management of patients with type 1 diabetes.
The inventors called the system a 3D bioprinter for pancreatic cell transplantation (PICT). The new technology was presented to the Minister of Health of South Australia and then transferred to the Royal Adelaide Hospital, which became the first clinic in the world with such equipment.
3D Bioprinter
The developers explain that the system applies a special bioink containing insulin-producing islet cells to transplantable 3D printed scaffolds. This method is expected to improve the current process of islet cell transplantation from human donors used to treat severe cases of diabetes. The new technology reduces the risk of rejection of the transplanted tissue by incorporating the patient's cells into the donor tissue.
The PICT bioprinter will allow us to uniquely mix donor cells with recipient cells and create new complex “organoids” for experimental transplantation,” explained Professor Toby Coates. |
In addition, the bioprinter prints several types of cells, so its scaffold structure can also include endotheliocytes necessary for the growth of new blood vessels in the transplanted islet tissue.
The Research Council has awarded a grant to the Australian Center of Excellence in Electrical Materials, chaired by Professor Gordon Wallace, and his team will now further develop and improve the 3D bioprinter delivered to the Royal Adelaide Hospital.
Together with the team of Toby Coates at the Royal Adelaide Hospital, we plan to improve the efficiency of islet cell transplants by embedding donor materials in a 3D printed structure to protect them during and after transplantation,” said Professor Wallace. |
3D printing of the middle ear to restore hearing
At the annual meeting of the Radiological Society of North America (RSNA) in December 2017, it was shown how 3D printing can be used to reproduce exact copies of the middle ear to restore hearing to people. The development began to be put into practice.
By converting 3D CT scans into 3D printed prostheses, surgeons have successfully placed four implants of varying sizes into human ears.
| Assuming that the most likely cause of failure with an existing prosthesis is an improper fit, then being able to create a custom prosthesis that can accurately fill an air-bone gap is the least likely to result in failure, says study author Dr. Jeffrey Hirsch ( Jeffrey Hirsch, assistant professor of radiology at the University of Maryland at Baltimore, in an interview with HCB News. “Our study shows that even at the submillimeter level, there are tiny differences in different ears that can be accurately reproduced using 3D modeling. Visual comparison of coin size and 3D printed middle ear prosthesis According to the scientist, this method can improve the surgical procedure, which often fails due to incorrect sizes of prosthetic implants. In this study, four surgeons performed implant placement in four different middle ears. All surgeons were able to accurately align the prosthesis model with the temporal bone containing the middle and inner parts of the ear. Hirsch explained that the ability to see complex anatomical relationships in 3D modeling allows for a new level of study, understanding and medical planning. The next step for researchers will be to develop a biocompatible material. As such a platform, a group of researchers considers the use of cultured stem cells. FDA Guidelines for Medical 3D Printing On December 4, 2017, the US Food and Drug Administration (FDA) issued new guidelines for creating medical models using 3D printers. The manual discusses in detail aspects of the design and testing of models, as well as requirements for their quality. Although 3D printing is a relatively new technology, it has already found widespread use in clinical practice, such as replicating complex anatomical structures and simulating surgical procedures. Noticing the rapid evolution of this technology, the FDA has issued specific guidelines to help manufacturers bring 3D printed models to market more efficiently.
Example of 3D printed part of the skull Based on the August 31, 2017 FDA Joint Seminar with the RSNA 3D Printing Expert Panel, the recommendations focus on the technical aspects of 3D printing. Recommendations include sections on design and manufacturing process, model testing, and instruction writing. The manufacturing process section covers the technical aspects of 3D printed models. When creating models based on images, such as those obtained from CT scans, consideration should be given to the minimum image quality and resolution, image processing algorithms that can change the dimensions of the model compared to real organs, and the preservation and definability of anatomical landmarks used for adaptation. models. Model testing section provides requirements for model description, mechanical test results, dimensional measurement, material characteristics, sterilization and biocompatibility. According to the third section, each device must have instructions that will indicate the patient ID, the purpose of the model and its final design, as well as a warning about the need for a preliminary examination of the patient to rule out any changes that may distinguish the model from the real anatomical structure. Mobile Infection Detector Print In October 2017, a group of American engineers and scientists developed a new "on-the-spot" infectious disease diagnostic system that uses a conventional mobile phone and a credit card-sized diagnostic chip as a detector. The solution was created using 3D printing technologies.
3D printed mobile infection detector Low cost, portability, as well as the use of a conventional mobile phone as a detector, makes this diagnostic complex indispensable for diagnosing infectious diseases in conditions of limited resources or when the diagnostic result is needed immediately. Integration of the diagnostic platform with modern mobile communication systems will allow for personalized treatment of patients and monitoring of the epidemiological situation. At the same time, the time for obtaining diagnostic results is comparable to the time for conducting similar tests in a stationary laboratory - about 30 minutes. The complex itself consists of an ordinary smartphone and a portable 3D-printed cradle socket containing optoelectronic "stuffing", as well as a special interface for the smartphone's camera. The application running on the smartphone collects the results of the tests carried out using the microfluidic chip and patient data, which are then transferred to the cloud database. During demonstration tests, the complex was used for the qualitative and quantitative analysis in blood drops of infections that cause respiratory diseases in horses - Zika fever, Dengue fever and Chikungunya fever. Robotic arm replacing sign language interpreter In August 2017, the media reported on the development of graduate students from the University of Antwerp (Belgium), which can make life easier for deaf people. Sign language interpreters are often in short supply, which is why it was decided to create an inexpensive automated system that can translate text into sign language.
3D printed robotic arm to replace sign language interpreter
The robotic arm assembled by the inventors consists of 25 3D printed plastic parts and is powered by 16 servos controlled by the Arduino platform, reports Tech Crunch. The developers are planning a system with two robotic arms and a face to convey emotions. So far, only a prototype of the device exists, but enthusiasts intend to complete the project and make the materials of their work publicly available so that those who wish can make their own sign language interpreter. Artificial heart seal In July 2017, the Swiss Federal Institute of Technology Zurich (ETH Zurich) unveiled a 3D printed artificial heart. An artificial heart weighing 390 grams and with a volume of 679 cubic centimeters is 3D printed using investment casting. The left and right ventricles are not separated by a septum, but by a special chamber filled with compressed air. Inflating and deflating, this chamber imitates the contraction of the muscles of the human heart and pumps blood. At the time of the demonstration, the artificial heart only supports 3,000 beats, which means it can work from 30 to 45 minutes. To test the work of the heart, scientists used an advanced test environment that mimics the human cardiovascular system, and a fluid that has a viscosity comparable to blood. The operation of the device was captured on video.
By 2017, about 26 million people suffer from heart failure. Most of them are hopelessly waiting for donors to provide them with a new heart. These patients are fitted with special blood pumps that facilitate the work of the heart, but they can cause serious complications and do not provide patients with a pulse. Seal of ovaries In May 2017, ovaries were 3D printed that allowed infertile mice to give birth. Scientists intend to test the development on humans. Scientists at Northwestern University Chicago have created an artificial ovary that allows full restoration of reproductive function. During the experiment, an infertile laboratory mouse was implanted with a prosthesis created using 3D printing. Subsequently, the mice (three out of seven) were able to feed on their mother's milk and get healthy litters. Bioprosthetic ovaries consist of a porous scaffold of gelatin ink that is filled with follicles, tiny fluid-filled sacs that store immature eggs. The recipient mouse body actually coordinated the development of ovarian tissues, and blood flow through the pores helped turn the implanted structure into a functional bioprosthesis. However, it is worth noting that not the entire ovary was printed, as it is too complex an organ. Scientists created the connective tissue basis of the ovary: the printer was charged with gelatin, which was obtained from collagen, one of the main proteins of connective tissue - collagen was in the form in which it is usually present in the ovaries of animals. Then, mouse follicles with eggs inside were immersed in the resulting (printed) gelatin base. It is not yet clear whether such a prosthesis will fit a human, as female follicles are much larger and grow faster. However, scientists promise to conduct research aimed at developing the idea in a human direction.
3D printed heart on chip At the end of October 2016, researchers at Harvard University announced the creation of the world's first 3D-printed heart on a chip. The new development will allow experiments related to the work of the heart to be carried out without the participation of experimental people and animals, the university website says. The results of the study itself, conducted by scientists from the Harvard John A. Paulson School of Engineering and Applied Sciences and Applied Sciences and the Institute of Biotechnology. Visa (Wyss Institute for Biologically Inspired Engineering), published in the journal Nature Materials. The heart on a chip is made of a translucent synthetic material that mimics the structure and function of the heart tissue. A 3D-printed organ cannot serve as an implant for a person, but is intended only for scientific research. Thanks to the new technology, it will be possible to reproduce hereditary diseases in the laboratory with the reconstruction of the cells of a specific patient, as well as to test various methods of treatment on artificially grown tissues in order to choose the most effective one. Creation of 3D models of organs before surgeries in Dubai In October 2016, it became known that 3D printers will appear in medical institutions in Dubai, printing accurate models of the organs of patients to be operated on. Thanks to the new technology, it is planned to improve the accuracy and efficiency of surgical operations. According to Gulf News, all hospitals under the control of the Dubai Health Authority (DHA) in the United Arab Emirates (UAE) will be equipped with equipment for 3D printing of prosthetic limbs and teeth that mimic cast fractures and human models. organs to simulate operations before direct surgical contact with the patient.
Dubai surgeons to train on 3D organ models before operations According to Sheikh Mohammed bin Rashid Al Maktoum, Vice President of the United Arab Emirates and Ruler of Dubai, this initiative will speed up medical procedures, cut costs and help doctors plan complex surgeries.
Regulators in the UAE are working to keep laws in line with the rapid development of 3D printing technology, he said. The use of 3D printers for medical purposes has become part of Dubai's 3D printing strategy, which aims to make the city a leading hub for the technology by 2030. All new buildings in Dubai are expected to have 25% 3D printed parts by this point. Creation and implantation of a 3D skull In April 2016, it became known that South Korean surgeons were able to print a skull model on a 3D printer and use it on a living person. The operation was successful and helped save a human life, according to a publication on the 3Dprint.com website. A 60-year-old female patient was admitted to the Chung-Ang University Hospital in South Korea with a sudden headache. She was diagnosed with a subarachnoid hemorrhage. After trying in vain to stop the fatal bleeding, doctors decided to remove part of the skull to relieve the pressure on the brain caused by swelling.
3D printed skull successfully transplanted in South Korea During an operation, the blood supply to the brain failed at the site of the removed part of the skull, as a result of which a skull transplant was required. As a result, it was decided to implant a three-dimensional model of the skull. Doctors from the hospital recruited specialists from the Korea Institute of Industrial Technology in Gangwon Province. They scanned the patient's skull using computed tomography and created an exact three-dimensional copy of the organ. Using special equipment, the model was printed. It was made from pure titanium, which is considered to be one of the best materials for creating implants. This metal is light, strong and inert, it has a low probability of rejection by the body. The 3D-printed skull has been successfully implanted. Kwon Jeong-tek, professor of the Department of Neurosurgery at Chunan University, noted that the creation of synthetic implants and metal plates used to connect bone fragments has long been used to replace elements of the human skull, but this technology has always been imperfect. Medical 3D printing software In a webinar hosted by the Society for Imaging Informatics in Medicine (SIIM) in late March 2016, University of Utah Dr. Justin Cramer listed the main software products that can be used for 3D printing in medicine.
3D printed prosthetic arm
When choosing software for 3D printers, Justin Kramer recommends a simple rule: for beginners, free options are fine, but if you plan to create accurate anatomical models for professional use, then it is better to purchase a powerful paid product, since it can be used to create a better model. University of Utah Developments: Cheap 3D Fusion Printing At the end of March 2016, the Society for Imaging Informatics in Medicine (SIIM) held a webinar during which radiologists from the University of Utah spoke about the capabilities of their new 3D printing laboratory. Its feature is the use of inexpensive equipment. Fused deposition modeling (FDM) has been selected for 3D printing. The technology involves the creation of three-dimensional objects by applying successive layers of material that follow the contours of a digital model. 3D printed model of the spine (left) According to Edward Quigley, Ph.D. from the University of Utah, the fusing method is a versatile and cheap way to create three-dimensional objects, which is why it is often used to develop entry-level medical 3D printers. The University of Utah has built a low cost FDM printer that can print fragile and complex anatomical models used for educational purposes. However, there were also successful experiments at the university. One of them is shown in the illustration above. In the picture on the left, you can see a 3D printed nylon model showing the cervical vertebrae, vertebral arteries, dural sac and spinal cord. On the right is the virtual version from which the physical prototype was created. Edward Quiglin noted that 3D printing can be used for research, intraoperative planning of operations, in cardiovascular and pulmonary surgery. Such technologies are especially useful in traumatology, and can also be used, for example, to create a guide for a biopsy needle or a guide sleeve for drilling teeth, he added.
Source
|
Additive technologies in medicine, 3D printer, bionic eye, robotic arm prosthesis, 3D printer for printing tissue from sugar for growing organs and studying tumors, 3D model, 3D bioprinter
Attention!
We accept news, articles or press releases
with links and images. [email protected]
Bioprinter: 3D printing of organs
3D bioprinting is an advanced technology that saves lives and helps create new medicines. What is the peculiarity and complexity of developments, who is engaged in them and what successes have already been made - this will be discussed in the material. We will also get acquainted with all the methods of bioprinting known today.
- How bioprinters print and what is 3D bioprinting
- The first biological 3D printer
- 3D bioprinting technologies
- Multimaterial Multinozzle 3D (MM3D) Technology, Wyss Institute
- Sound-Induced Morphogenesis (SIM) Technology, mimiX Biotherapeutics
- Biopixlar Bioprinter, Fluicell
- CELLINK Bioprinters
- Bioprinter that creates micro-human ears for patients University of Wollongong
- Bioink
- 3D Bioprinting Solutions
- What do 3D bioprinters print?
- Where do they print?
- 3D bioprinters in Russia
How bioprinters print and what is 3D bioprinting
3D bioprinting is the creation of 3D models using biomaterial, which includes living cells. It is used to reproduce complex structures such as skin tissue or blood vessels.
Model cells are taken from the patient and cultured until their mass is sufficient to create a bioink. The resulting ink is loaded into the printer, which prints the desired model.
Getting enough cells is not always possible, so seaweed or porcine collagen protein comes to the rescue. Stem cells are also used, which have the ability to become any cell in the body.
The first biological 3D printer
The first mass-produced bioprinter was released by the American company Organovo by the end of 2009. The Australian company Invetech became its industrial partner. Thanks to joint efforts, the machine was born, which in 2010 printed the first full-fledged blood vessel.
Organovo representatives decided to move away from the idea of growing organs in a test tube and suggested that it would be much more efficient to print it. They came up with the NovoGen technology, which regulated all the interactions between the biological component of the process and its mechanical part. Invetech was involved to implement the idea. The collaboration resulted in a compact device with an intuitive interface.
The printer had two printheads. One was filled with the necessary biomaterial, the second - with auxiliary components (collagen supporting the hydrogel, growth factors). Printing accuracy reached micrometers, which played an important role in the correct placement of cells.
3D bioprinting technologies
There are several technologies and approaches being developed and applied in the field of bioprinting. Scientists and researchers from private companies and institutions work on each of them.
Technology Multimaterial Multinozzle 3D (MM3D), Wyss Institute
The technology developed at the institute is based on the use of fast moving high pressure valves. The applied method makes it possible to switch between materials up to 50 times per second. This speed is faster than you can see with the naked eye.
The printheads themselves are 3D printed, so they can be easily customized to your specific needs.
![]()
The technology is suitable for the manufacture of complex objects, including moving robots. The method significantly speeds up the creation of complex models, because the printheads can use several nozzles at once.
Sound-Induced Morphogenesis (SIM) Technology by mimiX Biotherapeutics
Swiss technology is based on the reproduction of well-defined biological patterns that self-assemble into functional tissues using sound waves. The method embodies a highly efficient sequence for reproducing organized and dense cellular structures.
The mimiX technology uses sound waves. The space for growing cells is formed around a given type of speaker. Depending on the shape of the cup and the sound produced, structures of various shapes, such as gratings, are formed.
The creation of this method serves an important purpose - the availability and speed of playback, which can be achieved in any room. Prior to the invention of the SIM, bioprinting was available for scientific research. For clinical, it has become too complicated and lengthy. Now this has changed.
Fluicell Biopixlar Bioprinter
Swedish-based Fluicell focuses on creating platforms for studying cell behavior. Her method of work makes it possible to make complex structures that mimic tissue, in which the location of individual cells is controlled by a gamepad. The workflow is similar to a video game.
The company uses the micro-jet technique. Thanks to the micro jet tube and the precision of the pump during the direction of the biomaterial to the printing zone, it gives micro-level control over the material. Because of this, systems scale to the macro level naturally. High resolution prints are produced.
The method makes it possible to reproduce multicomponent structures, while the material can be created in the printer itself. This approach eliminates the need for laboratory preparation. The mixing progress of different materials is controlled in the microfluidic chamber. The result is a 3D printed finished structure, which was created without the use of gels and scaffolds.
The technical capabilities of this method make it ideal for processing scarce materials such as biopsy specimens, stem cells and primary cells.
CELLINK Bioprinters
CELLINK is a Swedish company that develops bioprinting technologies for applications in various fields, including cosmetics and medicine. The principles generated by the organization work to create skin tissue, cartilage, liver and other products.
In 2019, the manufacturer launched two models of bioprinters on the market - Bio X6 and Lumen X. The first one is designed for creating constructs with any type of cells. With it, you can reproduce any tissue found in the body. The company focuses on quick results and combining a large number of materials.
The Bio X6 has 6 printheads built in with CELLINK Clean Camera Technology. It is equipped with a smart interchangeable head method and two powerful fans to create excess air pressure inside the chamber. The user gets the opportunity to combine several materials in one print with a structure of increased complexity.
The second Lumen X unit is the result of a collaboration with the American company Volumetric. This is a startup focused on creating bioprinters using SLA technology. The device has a low cost and modest dimensions. At the same time, it has high printing accuracy and excellent performance. Such characteristics are especially important for the creation of vascular structures. Lumen X gets the job done 10 times faster than its competitors under the same conditions.
Bioprinter for Microtia Patients Creating Human Ears, University of Wollongong
Myctoria is a congenital defect associated with developmental arrest of the outer ear. It is found during pregnancy. Since the structure of the ear has a specific shape, the treatment of its deformity causes serious difficulties. According to the creators of the bioprinter, the new technology is leading to a revolution in helping children with microtia.
The Australian University of Wollongong created the Alek 3D printer. It prints human ears for further use in reconstructive surgery. Stem cells serve as the basis for bioink. The creation of ears on a bioprinter looks very promising, since the method allows you to design a transplant according to the shape of the patient's face and do it in a fairly short time. The technology excludes the search for donors to take a piece of cartilage, the work is based on the use of the patient's natural tissues.
Bio-ink
Manufacturers are engaged not only in the development of technology and design of printing devices, but also in materials that can be used to create complex structures and entire organs.
Allevi Liver Tissue Ink
The complexity of reproducing the liver lies in the fact that it has many important functions for life. There are more than five hundred of them. A small number of manufacturers are able to create bio-ink for the liver that will meet all the necessary conditions.
The American company Allevi is one such company. The drug, which makes it possible to reproduce tissue-like structures that mimic the natural characteristics of tissues, can be freely purchased through the Allevi online store.
Biogelx Synthetic Bioink
Biogelx is a company from Scotland. It is based in a laboratory at the University of Strathclyde in Glasgow. The direction of the company is the research and creation of artificial materials for bioprinting.
Hydrogel ink has a unique chemical and physical variability. It allows you to accurately recreate a variety of tissue parameters. As a result, cells are able to interact in an almost natural environment.
Ink helps keep cells alive. They provide a simple crosslinking technique and viscosity control as well as a high degree of reproducibility. Due to its positive technical abilities, the material is compatible with a wide range of 3D bioprinters.
Hydrogel with mineral nanoparticles, University of Texas
American development from the staff of the University of Texas (TAMU). Scientists have created a material in the form of a hydrogel. It contains mineral nanoparticles. They have the ability to carry out protein preparations to control the behavior of cells. Inks of this kind help in the field of creating tissues containing blood vessels.
Developers have been developing hydrogel bioinks based on the inert polymer PEG (polyethylene glycol). Printing with this type of ink is difficult due to its low viscosity. In the course of research, it turned out that silicate nanoparticles effectively increase the degree of viscosity, while almost not changing the other technical parameters of the printed material. Thus, TAMU workers have created a new class of hydrogels.
Bio-ink for artificial leather printing, Renseller Polytechnic Institute
The production of high-quality skin imitations after burns and other wounds has occupied the minds of bioengineers for many years. There are 2 ways to treat serious skin lesions. The first is the use of autologous skin grafts. Healthy tissue is taken from the patient and transplanted to damaged areas, while fresh wounds are formed and, in general, the procedure is quite unpleasant and painful.
The second method is to use skin substitutes made from foreign materials, such as bovine collagen. Such imitations do not completely cover deep wounds and are very different from natural skin.
Skin resurfacing technology from a collaboration between Rensselaer Polytechnic Institute (RPI) and Yale University promises to revolutionize skin grafting. They created bio-ink using living human cells. The material helps in reproducing artificial skin, which later itself recreates the system of blood vessels.
3D Bioprinting Solutions
3D Bioprinting Solutions is the only bioprinting company in Russia. The company was founded in 2013. One of the co-founders was the co-founder of INVITRO - Alexander Ostrovsky. His specialty is a resuscitator.
In 2014, the laboratory presented the first bioprinter made in Russia.
He received the name FABION. According to the list of the use of various printed materials, this device is one of the leaders in the field of multifunctional devices.
In early 2015, the company created and successfully transplanted a mouse thyroid organ construct. In 2016, researchers produced a printhead that can automatically feed tissue spheroids for 3D bioprinting. The development was applied in the new version of the device - FABION-2.
After the release of an updated version of the device, the company's specialists focused on creating a printer that uses a new working methodology, different from previous solutions. It was the principle of magnetic levitation and the ability of microtissues to self-assemble from tissue spheroids. A fully functioning magnetic assembly saw the light of day by the spring of 2017.
At the end of the summer of 2017, cooperation with the state company Roscosmos began. The laboratory has signed a contract for the implementation of a space biofabrication experiment aboard the Russian part of the ISS. Scientists have begun to develop the Organ.Avt printer capable of working in zero gravity.
The Magnetic Bioprinter experiment started at the end of 2018. In its course, models of human bone and cartilage tissue, as well as the mouse thyroid gland, were printed. To carry out the work, the Soyuz MS-11 crew members underwent appropriate training at the 3D Bioprinting Solutions laboratory.
In addition to bioprinters, the company has developed a line of multifunctional collagen products - Wiscoll. It is suitable for use in any 3D bioprinter. Bioink is used for a wide range of biofabrication experiments. The product is a concentrated solution of collagen of the first type of the highest degree of purification. It is immediately ready for use.
What is printed on 3D bioprinters?
Printing Organs
Printing fully functional complex internal organs is not yet possible, although research is ongoing in this area. For example, the bladder has already managed to reproduce. It happened in 2013 in the USA (Wake Forest University).
Scientists have extracted raw material from a patient's poorly functioning organ, nurtured them and added nutrients. Next, they reproduced the shape of the bladder according to the parameters of the patient and cultured cells impregnated through it. The model was placed in an incubator, brought to the required condition and transplanted into a human. Over time, it collapsed, leaving completely organic material in its place.
The same team produced viable urethras. Research is ongoing and breakthroughs are being made in the creation of the kidneys, liver and heart.
Printing tissues and vessels
The human body is pierced by tens of thousands of kilometers of capillaries, arteries and veins. Over time, they wear out, and scientists are conducting research on the possibility of their full replacement. Creating body parts on a bioprinter is impossible without reproducing viable blood vessels. Technologies make it possible to create materials designed for further favorable independent development of blood vessels.
Surgical practice
Surgeons now have the opportunity to practice performing operations on organs and tissues that look 100% real. This happens both with the help of virtual prototyping and with the use of 3D printed models.
New drug testing
Bioprinted tissue has several cell types with different densities and key architectural features. This makes it possible to conduct research on the impact of diseases on the body, as well as to work out various methods of treatment.
Where do they print?
Organizations that offer organ printing or sell bioprinters:
- 3D Bioprinting Solutions - Russia, Moscow. Specializes in frameless printing, created two printers - FABION and FABION-2. Develops his own method of organoprinting.
- Organovo - USA, San Diego. Produces and sells liver tissue to pharmaceutical companies.
In 2009, the first mass-produced bioprinter, Novogen, was released.
- BioBots - USA, Louisville. A startup that introduced a cheap bioprinter for commercial use in 2013. Available BioBot BASIC. Work is underway on the second version of the device.
- Cyfuse Biomedical - Japan, Tokyo. The company produced the Regenovo bioprinter, which can be used to create skin tissues and grow blood vessels.
3D bioprinters in Russia
So far, bioprinting devices in Russia are represented by only one company created by the co-founder of the INVITRO network - 3D Bioprinting Solutions. Ongoing research on the Russian part of MSCs under weightless conditions gives researchers hope that unique data will be obtained, on the basis of which new drugs will be developed.
Scientists are also optimistic about the creation of functional complex organs of the human body, saying that they will appear already in the current century.
Emerging technologies in the field of bioprinting make it possible to draw the following conclusions:
