Additive manufacturing technologies 3d printing rapid prototyping and
Origins of 3D Printing and Additive Manufacturing
Additive manufacturing, often synonymous with 3D printing, has received much attention in the last decade. However, this seemingly modern technology has, in actuality, been around for nearly 40 years.
Traditional manufacturing involves machining, a subtractive process, in which material is removed in a controlled manner to produce the desired shape. This is achieved through various techniques including milling/cutting, turning, drilling/boring, etc. Modern machining almost always uses computer numerical control (CNC) tools. In contrast, 3D printing is an additive process in which material is gradually layered to produce the desired form. There are multiple techniques and technologies in use today, the end product and material help determine which method of additive manufacturing should be used. One thing that all additive manufacturing techniques have in common is their origins.
The concept of producing a 3-dimensional structure by layering material can be traced back to 1892 with J E Blanther’s wax sheet topographical maps concept, but this is a far cry from the 3D printing we know today. It would not be until 1981 that automatic methods for producing 3D models would be detailed by Hideo Kodama. Kodama’s published paper described three techniques for forming 3-dimentional plastic models using ultraviolet (UV) cured layers of photopolymer material. A few years later, in 1984, a patent would be filed by Chuck Hull coining the term stereolithography, a technique utilizing UV light lasers and a bed or vat or photopolymer resin to produce 3D objects. In 1986, the first 3D printing company in the world would be co-founded by Chuck Hull, brining additive manufacturing to the commercial market.
Today there are many additive manufacturing technologies; ISO / ASTM52900 “Standard Terminology for Additive Manufacturing” defines additive manufacturing into 7 technological categories: Vat photopolymerization, binder jetting, material extrusion, powder bed fusion, directed energy deposition, material jetting, and sheet lamination. In addition to these 7 categories, there are multiple proprietary technologies, some examples include: Fusion Deposition Modeling (FDM), a form of material extrusion, trademarked by the Stratasys Corp. and Direct Metal Laser Sintering (DMLS), a form of powder bed fusion, trademarked by Electro Optical Systems GmbH. The following paragraphs contain a summary of the 7 additive manufacturing categories that are frequently referenced.
Vat Photopolymerization
The most popular form of vat photopolymerization is Stereolithography or SLA. In SLA 3D Printing, the build platform is positioned in a tank (or vat) of liquid material that is gradually exposed to the UV laser, one layer at a time. The UV laser cures and hardens the liquid photopolymer into the desired shape as the workpiece is raised out of the fluid bed.
Binder Jetting
Sometimes referred to as Inkjet 3D printing, binder jetting uses a moving nozzle head to selectively deposit liquid binder on a powder bed. The workpiece is gradually formed as the build platform is lowered and additional powder is layered over the workpiece. The process originally used starch and plaster for the powder and water as the binding agent. Since then a wide range of materials from, sand to ceramics and even metal.
Material Extrusion
One of the most common forms of material extrusion is Fused Deposition Modeling (FDM). FDM is a material extrusion technology in which molten thermoplastics are deposited layer by layer to create the desired form. A secondary material must be used to form a support structure for more complicated or overhanging elements, as this method does not use a bed of material which inherently supports itself. Fused deposition modeling is the dominant technology behind the lower cost hobbyist machines now on the market.
Powder Bed Fusion
Selective Laser Sintering falls into the family of powder bed fusion. This process uses heat energy to fuse materials such as metal or ceramic powders into prototypes and functional products. It was invented at the University of Texas and became the company DTM which was eventually bought by 3D Systems. A laser is used to heat the powder which is usually preheated in bulk by the SLS machine to just below its melting point. The model is held in place by the unfused powder, avoiding the need for supporting struts that are common to other additive manufacturing methods. Sintering is a misnomer as the material is fused or melted, not compacted as in traditional sintering operations.
Directed Energy Deposition
Much like powder bed fusion, the direct energy deposition technique uses heat energy to melt mainly metals and can produce functional parts in addition to prototypes. The process is used to manufacture larger parts than possible with powder bed fusion albeit with inferior finishes. Post-build machining is generally required with directed energy deposition techniques.
Material Jetting
This additive manufacturing technology is most similar to traditional 2D printers in that material is deposited from an inkjet printhead without a bed of material or vat. Droplets of thermoset photopolymers are deposited onto the build platform, cured by UV light, and built up line-by-line, layer-by-layer. Similar or synonymous material jetting processes include Multijetting, Polyjetting, and Drop on Demand.
Sheet Lamination
Also called Laminated Object Manufacturing, this form of rapid prototyping uses sheets of paper, film, etc. which are laser cut and bonded to preceding layers. The model is then “excavated” from the laminated stack.
The term Rapid Prototyping entered the lexicon as a result of the initial applications of additive manufacturing. Early 3D printing was primarily used to make scale models and functional prototypes. The need to quickly test parts without cutting into production times created a niche market for those first companies to commercialize the process.
Rapid prototyping has been dominated by service providers who could afford the machinery and get enough prototyping work to make their investments pay off. Patents for most additive manufacturing processes has limited open-source exploitation of the various technologies. Some of that is changing with patents expiring but it remains primarily a tool for manufacturing parts in small lots and as a design aid to test prototype products for fit, ergonomics, function, etc. before they are brought to production runs.
The introduction of so-called personal 3D printers created a stir some years back, which many attribute to the expiration of the original Stratasys patents covering fused depositional modeling. Indeed, many of the consumer level machines use plastic filament as the printing material. The fact that ASTM recently defined standard terminology for additive manufacturing technologies may be a hat tip to its anticipation of a coming wave.
There are bona fide applications of additive manufacturing that cannot be duplicated economically by traditional manufacturing techniques. Parts can be made with complex internal geometries that would be prohibitively expensive to manufacture by lost wax casting, for instance. The jewelry industry is one such industry that has long relied on investment casting but is now turning to additive manufacturing. Other industries look to additive manufacturing to make custom medical implants or lightweight, low-volume aircraft parts. 3D printers are even in use on the International Space Station. Some manufacturers anticipate being able to reduce inventories of spare parts for their existing products and be able to support otherwise obsolete equipment by maintaining STL files and printing parts on demand.
3D printing has taken the stage as a poster child of the future of manufacturing. Although there are still many setbacks to widespread use, the main issue ends up being the economy of scale. However, as the cost of additive manufacturing technologies decreases and fidelity increases, and as long as it is still viewed as a viable technology of the future, additive manufacturing will become a production manufacturing staple with rapid prototyping always at hand for initial product development.
References
- How 3D Printing Works - Xometry
- http://www.3dpmuseum.com/
- http://www.
astm.org/cgi-bin/resolver.cgi?ISOASTM52900
- http://en.wikipedia.org/wiki/Stereolithography
- http://en.wikipedia.org Powder bed and inkjet head 3D printing
- http://en.wikipedia.org Selective laser sintering
- https://www.additively.com/en/learn-about/binder-jetting
- http://en.wikipedia.org Laminated object manufacturing
- http://3dprintingindustry.com/2013/12/29/many-3d-printing-patents-expiring-soon-heres-round-overview
- https://www.space.com/33166-space-station-commercial-3d-printer-first-tool-photos.html
- https://www.chaparraltech.net/what-is-additive-manufacturing/
Other 3D Printing Articles
- Top 3D Printing Services Companies in the USA
- Overview of 3D Printing Technologies
- Top 3D Printing Manufacturers and Suppliers (Publicly Traded and Privately-Owned)
- Top Suppliers of Additive Manufacturing Consulting Services
More from Custom Manufacturing & Fabricating
“Additive Manufacturing Technologies: 3D Printing, Rapid Prototyping, and Direct Digital Manufacturing”, 2nd Edition
“Additive Manufacturing Technologies: 3D Printing, Rapid Prototyping, and Direct Digital Manufacturing”, 2nd Edition - technology.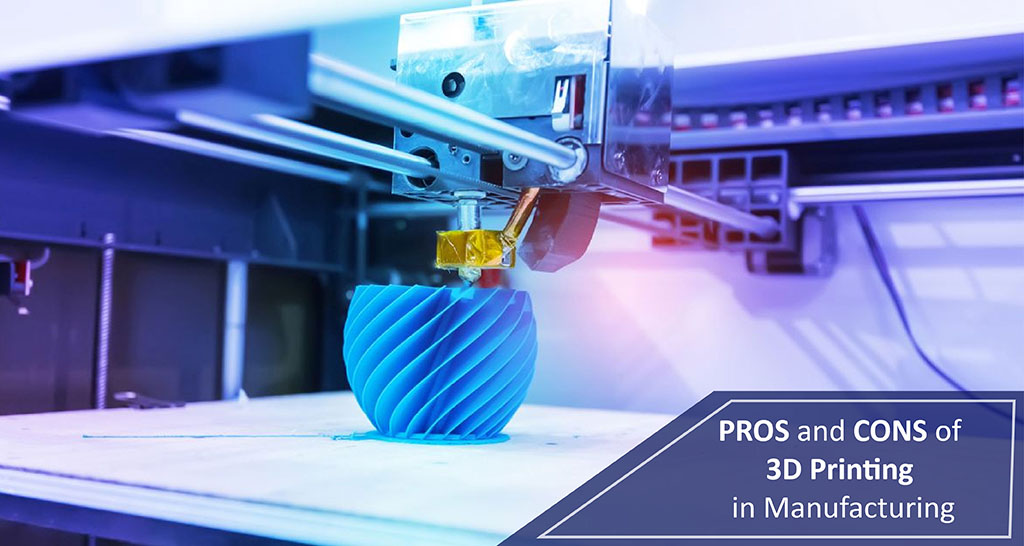
“Additive Manufacturing Technologies: 3D Printing, Rapid Prototyping, and Direct Digital Manufacturing”, 2nd Edition
English(Chinese)
Journal Archive
Johnson Matthey Technol. Rev., 2015, 59, (3), 193
doi: 10.1595/205651315X688406
“Additive Manufacturing Technologies: 3D Printing, Rapid Prototyping, and Direct Digital Manufacturing”, 2nd Edition
By Ian Gibson (Deakin University, Australia), David Rosen (Georgia Institute of Technology, USA) and Brent Stucker (University of Louisville, USA), Springer Science+Business Media, New York, USA, 2015, 498 pages, ISBN: 978-1-4939-2112-6, £81.00, €96.29, US$119.00
Share this page:
- Digg
- MIX
Introduction
“Additive Manufacturing Technologies: 3D Printing, Rapid Prototyping, and Direct Digital Manufacturing” is authored by Ian Gibson, David Rosen and Brent Stucker, who collectively possess 60 years’ experience in the field of additive manufacturing (AM). This is the second edition of the book which aims to include current developments and innovations in a rapidly changing field. Its primary aim is to serve as a teaching aid for developing and established curricula, therefore becoming an all-encompassing introductory text for this purpose. It is also noted that researchers may find the text useful as a guide to the ‘state-of-the-art’ and to identify research opportunities.
The book is structured to provide justification and information for the use and development of AM by using standardised terminology to conform to standards (American Society for Testing and Materials (ASTM) F42) introduced since the first edition. The basic principles and historical developments for AM are introduced in summary in the first three chapters of the book and this serves as an excellent introduction for the uninitiated. Chapters 4–11 focus on the core technologies of AM individually and, in most cases, in comprehensive detail which gives those interested in the technical application and development of the technologies a solid footing. The remaining chapters provide guidelines and examples for various stages of the process including machine and/or materials selection, design considerations and software limitations, applications and post-processing considerations.
Principles and Processes
The first three chapters provide a basic understanding of why someone might want to utilise AM in the context of traditional methods as well as developments in AM since its inception. In the initial chapters the reason for introducing standardised terminology is justified in contrast to the historical terms. For example, the use of ‘rapid prototyping’ to describe the field is no longer relevant as the technology is now used for functional components as well.
Brief summaries of the process are also provided in these initial chapters in increasing detail as the reader approaches the technical chapters. This provides a realistic view of actions required throughout the process and gives context to references made throughout the book. In its simplest form the process can be universally summarised as:
-
conceptualisation and computer aided design (CAD)
-
conversion to STereoLithography (STL)/additive manufacturing file (AMF)
-
transfer to AM machine and file manipulation
-
machine setup
-
build
-
removal and cleanup
-
post-processing
-
application.
Additive Layer Manufacturing Technologies in Detail
Chapters 4–11 describe seven different printing processes in differing degrees of detail. Topics such as ‘vat photopolymerisation’ (VP) and ‘powder bed fusion’ (PBF) are covered in such comprehensive detail that their chapters are double the length compared with ‘binder jetting’ (BJ), despite the latter being a very well used industrial technique. Printable materials and delivery mechanisms are also listed here and the chapters include a ‘process benefits and drawbacks’ section, useful for the beginner. It should be noted that some processes (extrusion, sheet lamination and directed-energy deposition) are omitted from this review.
Vat Photopolymerisation
VP processes make use of liquid, light-curable resins as their primary materials. Upon irradiation, these materials undergo photopolymerisation, becoming solid. Ultraviolet (UV) light is projected onto the build plate, curing a layer before recoating. Methods for illuminating the photopolymers are presented, including masked projection, layer-wise processing and vector scan point-wise processing. Expressions relating laser power, scan speed, spot size and cure depth are derived, forming the basis of a process model which can be applied generally. Curable materials are discussed, including an overview of photopolymer chemistry and their interactions with radiation. Another method, called the ‘two-photon’ approach, describes how a dual light source can increase part resolution – feature sizes of 0.2 μm have been achieved. Advantages of VP include part accuracy, surface finish and process flexibility. The main drawback is their usage of photopolymers, which generally do not have the impact strength or durability of good quality injection moulded thermoplastics.
Powder Bed Fusion
PBF methods deposit layers of powder which are sequentially fused together by an energy source resulting in solid parts residing in a powder bed. The prominent methods are selective laser sintering (SLS) and electron beam melting (EBM), and these two are well compared: EBM processes benefit from the flexibility and high energy of their heat source, which can move nearly instantaneously and with split beams; EBM builds typically maintain the bed at high temperature and can create parts with a cast, low porosity, low residual stress microstructure. However their requirement for a vacuum chamber and conductive target material restricts material capabilities whereas SLS machines can process materials such as polymers and ceramics in gaseous atmospheres. This chapter builds on the process model from the previous chapter, applying the concepts to the fusion process of solids and methods for reducing internal stresses in solidified parts. PBF is a well commercialised technology, but due to the lapsing of major patents there are some open-source machines available which have led inventors to create non-engineering applications of the technology too.
Materials Jetting
The first generation of materials jetting (MJ) machines was commercialised in the 1980s. They relied on heated waxy thermoplastics deposited by inkjet printheads, lending themselves to modelling and investment casting manufacture; however the more recent focus has been on deposition of acrylate photopolymers, wherein droplets of liquid monomer are formed and then exposed to UV light to initiate polymerisation. Machines with build spaces as big as 1000 × 800 × 800 mm are available, as well as multi-material capability which can print 1000+ materials by varying the composition of several photopolymers. Research groups around the world are working on other material groups such as non-photopolymers, ceramic suspensions and low melting point metals (including aluminium). The challenge of forming molten droplets, then controlling deposition and solidification characteristics has kept these material systems in the research arena. A section on the fluid mechanics of droplet formation and jetting is included in a process model for the MJ process (a section which is also relevant to Chapter 8).
Binder Jetting
BJ methods were primarily developed at Massachusetts Institute of Technology (MIT), USA, during the early 1990s and feature a powder bed similar to that of the PBF process (Chapter 5). However, instead of using an energy source to fuse the material together, an inkjet head deposits a binder onto each layer to form part cross-sections. The binder forms agglomerates with the powder particles and provides bonding with the layer below. The chapter describes some commercial materials available, and notes that many of them require post-processing to increase strength, for example by infiltration with another material. Materials include polymers (for example, poly(methyl methacrylate) (PMMA)), ceramics, foundry sands and metals such as 420 stainless steel or Inconel alloy 625 infiltrated with bronze. This technology can be scaled up quite easily, as demonstrated by machines with an incredible 4 × 2 × 1 m build volume. Although the subject of ink drop formation is covered in the previous chapter, a discussion surrounding ink interaction with the powder bed is missing here. Disappointingly for a process described as one of the most readily scalable, the chapter is one of the shortest – although some aspects, such as powder handling, are discussed in other chapters.
Guidelines for Process Selection and Ancillary Process Tasks
The remainder of the book provides outlines and information on software applications and physical processes necessary for gaining maximum advantage from AM.
Process Selection
There are software applications where the performance attributes of each AM technology option are weighted and ranked based on the relevance of the input parameters (for example geometric considerations and mechanical properties). A particular example, RM Select, is provided (installation file and manual are available (1, 2)). This information is particularly pertinent to those interested in production applications of AM.
Post-processing
AM is often viewed as a complete process and therefore, to the uninitiated, it can be a surprise to learn of the requirement for post-processing. The book introduces the various types of support structures required to achieve and maintain all levels of geometric complexity and the subsequent post-processing methods and design considerations to aid their removal. Depending on the application of the part, surface finishing may also be required. In this case it may be necessary to design extra material in the areas to be post-processed to ensure the desired dimensions are maintained.
Powder bed methods possess ‘natural’ supports where the built object is encased in excess material. They are named as such as they are an intrinsic part of the process. The main disadvantage of natural supports is that the part must be designed so that the excess material can be removed, for example for powder bed methods parts that are designed to be hollow must have an escape hole for loose powder. In this case the excess material is recycled as much as possible with some sieving usually required.
‘Synthetic’ supports are required for methods that do not have ‘natural’ supports or for methods that have stresses as an intrinsic part of the process, for example powder bed fusion parts need to be tethered to the substrate to prevent warping from stresses created by the presence of significant temperature gradients. It is noted that these stresses can be reduced, and therefore the requirement for synthetic supports is decreased, by raising the temperature of the build environment. For MJ and materials extrusion methods the support structures can be constructed from the build material or a secondary material. In the case of secondary materials a second ‘printhead’ or a purge cycle is required to prevent contamination. If the ‘synthetic’ supports are constructed of the build material then they must be designed such that they can be easily removed in post-processing, it is common for printer software to include automatic support generation. Alternatively, the ‘synthetic’ supports can be constructed of a secondary material with differential solubility (with respect to the build material) and can simply be dissolved away post-process.
Post-processing is an integral procedure of AM and understanding the many factors to streamline and safeguard the integrity of the printed part is paramount.
Software Issues and Considerations
The file standard for AM technologies is the STL file format. The origin of this file extension hails to the first stereolithography machines commercialised by 3D Systems Inc, USA. This format is expressed as a list, either in binary or American Standard Code for Information Interchange (ASCII) (text), of the vertices of triangular facets used to approximate the surface of the digital part. This approximation is most relevant, with respect to deviation from the true geometry, when there are a significant number of curves in the part. The orientation of the facets is defined by the unit normally expressed in vector coordinates.
Due to the nature of the process, i.e. layer-by-layer, there is also the requirement to ‘slice’ the file prior to initiating the printing process. The generation of the support structures may be done before or during the slicing operation.
One problem with STL, unlike other CAD formats, is that there are no units associated with the file itself. Therefore the scale/dimensions must be checked to ensure the correct dimensions are applied. This can be particularly important for international communications, for example USA to EU or vice versa. Furthermore, it should be noted that not all graphics software packages can create STL files with adequate accuracy. One common issue when converting to STL is the creation of parts with holes (i.e. incomplete surfaces), which are not compatible with AM machines. More modern dedicated AM software packages are emerging, making this issue less prevalent.
Colour and materials properties are another factor to include into the definition of a part to print. For coloured parts this can be done by colouring of individual facets with solid colour (colour STL) or an image (virtual reality modelling language (VRML)). The advantage of applying an image is that the applied colour is not limited by the resolution of the facet. As the STL file format is surface data only there is the assumption that the underlying material is homogeneous. For AM technologies capable of multi-material printing the file typically has to be defined as distinct objects with materials properties defined for each. As yet, there is not an agreed upon standard file format for multi-material objects.
Design Considerations
Particular focus is made on the use of designed cellular structures and void filling. These structures can be symmetrical or conformal (with variable cell size to appropriately fill the void space). The primary advantage of using cellular structures is the reduction of the required build material without compromising overall strength. Cellular structures are a particular strength of AM processes given the fact that the entire structure can be designed, and with the help of software applications, optimised to achieve the most efficient strength-to-weight properties. The output cannot always be reproduced but a near approximation can be achieved with little compromise in performance. Examples of topology optimisation software given are Abaqus from Dassault Systèmes and OptiStruct from Altair.
Applications
This section offers a flavour of the multitude of possible applications of AM technologies. ‘Rapid tooling’ refers to the use of AM to create production tools. Typically these are reusable moulds, impressions or patterns from which the tools can be created. This method may be applied, in particular, if the material required for part production is not currently available in an AM technology or the mould can be improved upon by utilising the design freedoms of AM, for example conformal cooling channels for injection moulds. Medical and aerospace/automotive applications have received significant attention from the implementation of AM technologies. Medical procedures can be streamlined by developing surgical guides and tools. Prostheses and implants are of particular interest for the application of AM as they can be customised on a case-by-case basis. Significant research efforts are currently being employed to print with living cells and biomaterials for direct transplant. Another method is to print a biocompatible scaffold for post-process treatment with living cells to encourage natural material growth, for example Osteopore produces ‘bioresorbable’ implants to encourage natural bone growth over trephination holes from neurosurgery.
Aerospace applications of AM have been quite diverse with examples of engine system and non-structural components. The primary benefit for the aerospace industry is improved fuel efficiency by better performance and lighter weight components.
Business Opportunities and Future Directions
The book is rounded out by highlighting the fact that AM offers genuinely new avenues for production and product development and thus adjusted business models and practices could be required to accommodate the rapidly changing landscape of manufacturing.
Conclusion
Readers desiring a comprehensive introduction to the many technologies of AM should be satisfied. Although it is aimed primarily at students and educators, the authors do very well to appeal to those in research and manufacturing positions too. Excellent explanations of basic concepts through to the state-of-the-art make this a great starting point for in-depth research, whilst the process selection tools and business opportunities chapters will be very useful for manufacturers looking to explore this technology.
“Additive Manufacturing Technologies: 3D Printing, Rapid Prototyping, and Direct Digital Manufacturing” LINK http://link.springer.com/book/10.1007%2F978-1-4939-2113-3
References
- D. Rosen, ‘RM Selection, Software User Manual’, Version 1, Georgia Institute of Technology, Atlanta, USA, 12th August, 2005
- The Georgia Institute of Technology: The Systems Realization Laboratory: http://www.srl.gatech.edu/Members/drosen (Accessed on 28th May 2015)
The Reviewers
Jonathan Edgar received a BSc and PhD in nanotechnology from the University of Technology, Sydney, Australia. Currently he is working for Johnson Matthey on a core science and materials development project using additive manufacturing technologies.
Saxon Tint has an MEng in Materials Science and Engineering from Imperial College London, UK. He joined Johnson Matthey Noble Metals in 2011 as a Materials Scientist and has worked on a range of projects including alloy development for spark plug tips, powder metallurgy and AM.
Read more of this issue
Related articles
Flame, Combustion and Explosion Thermometry
Centralised and Localised Hydrogen Generation by Ammonia Decomposition
MORE ARTICLES
Find an article
ArticleSearchHow 3D printing complements traditional production
Analytics and business
Rapid prototyping
Experts recommend
Author: Andrey Kombarov
Author: Andrey Kombarov
From prototyping to production | Quality | Speed | Efficiency | Creativity | The evolution of additive manufacturing
We live in an era of rapid progress in technology and business processes, but we must admit that manufacturers are still limited by the eternal trinity: quality, speed and cost. Finding a balance between the two is not an easy task, but the good news is that the additive industry is reaching new levels. How exactly can 3D printing complement traditional manufacturing and change business models across industries? nine0003
According to Jabil's 2021 3D Printing Market Trends Survey, additive manufacturing is already helping manufacturers find new and unique approaches to better meet market demands. In recent years, regulated industries with strict safety and quality standards, including healthcare, aerospace, defense and automotive, have enthusiastically promoted the strategic benefits of additive manufacturing. nine0003
Digitalization will help manufacturers from different industries change the market, and not lose competitiveness in it. Both value metrics and the rapid expansion of digital manufacturing are pushing for the transition to digital technologies. Thanks to 3D printing, companies are better able to respond to changing conditions, complete joint projects and modernize.
The article discusses four key factors that underlie the interaction between additive and traditional manufacturing: nine0003
-
quality,
-
speed,
-
efficiency,
-
creativity.
Can 3D printing help you optimize production in your organization? Order a free consultation with iQB Technologies experts
nine0044 From prototyping to production
3D printing is usually associated with generating ideas, designing and prototyping, but it has long been cramped in this framework. Today, with the help of additive technologies, they create tooling, test products before launching into mass production, and manufacture small series of parts. According to Jabil's 2021 3D Printing Market Trends Survey of over 300 decision makers, 62% of companies use 3D printing for mass production. For comparison: in 2017, only 27% spoke about this. nine0003
From this we can conclude that distributed production networks are the reality of today. Global networks of additive manufacturing assets (such as the Jabil Additive Manufacturing Network) enable companies to manufacture products close to the point of delivery. The advantage of this model is "digital flexibility": one participant uploads design files in Chicago, while another prints them at a facility in Singapore, closer to the end customer. With this approach, supply chains are used much more efficiently. nine0003
Significant recent advances in the additive industry are setting the stage for distributed 3D printing manufacturing, accelerating progress and making the technology more practical. To list just a few of the aspects that define how 3D printing complements manufacturing.
1. Quality
3D printing allows manufacturers to produce unique, complex shapes of consistently high quality at a low cost. Thus, the additive method can advantageously produce a small number of parts of different sizes, and in some cases, one part of different sizes. nine0003
In recent years, the print quality and reliability of 3D printers have improved significantly, and they can now print more precise parts with better surface quality. In 2019, Jabil conducted a print quality survey. About 40% of the respondents answered that they were not sure about the reliability of the parts produced. Just two years later, 27% gave this answer.
In addition, new materials, platforms, and software enable companies to overcome the challenges of making parts repeatable from batch to batch and machine to machine. Thanks to these solutions, the company reduces the time and cost of organizing or reorienting production, which means it can respond faster to changing market requirements. nine0003
2. Speed
By using additive technologies combined with smart digital supply chains, virtual teams around the world can work on new projects, compare real product representations, save time along the concept-prototype-prototype-manufacturing chain, and even move production closer to customers. .
Compare this approach with the traditional one (involving investments in tooling, parts, equipment, partnerships and the release of the first production prototype) and see how obvious the advantages of additive manufacturing are: there is no doubt that it can affect all stages of product development. nine0003
Rapid prototyping provides the opportunity to explore different iterations and try out different designs during production. The healthcare, automotive and aerospace industries are undergoing rapid change, and 3D printing has become a key tool without which manufacturers cannot keep up with the most flexible and efficient product lifecycle management strategies.
Another important advantage of 3D printing is the ability to quickly change the range of manufactured products. Each printed item can be made unique, which means that the product can be created according to individual orders. Manufacturers can react faster to market changes and adapt production rates to demand. nine0003
As a result, additive technologies can reduce the time to market for new or upgraded products from months to days.
3. Efficiency
Additive-created products have simpler material specifications, which greatly simplifies supply chain management and speeds up production. An increasing number of businesses are using 3D printing platforms. Design improvements lead to more efficient design, lighter parts, and cleaner production, all of which contribute to an overall improvement in product performance. By simplifying specifications, not only the efficiency of the entire production process, but also the end product itself is increased. nine0003
Applying Design for Additive Manufacturing (DfAM) principles to create monolithic parts and system-level design results in merged specifications and reduced parts count, resulting in significant cost savings for companies and often increased product reliability.
3D printing has another economic advantage: various parts with complex geometries can be produced on a single 3D printer. Companies that have adopted the technology are able to save money by increasing the scale of production. The distribution of production along value chains is becoming more real. Rapid production of tooling, spare parts and more can all be done at facilities closer to the end customer, reducing costs all the way from transportation to warehousing. nine0003
Moreover, the digitalization of inventory management allows manufacturers to free up capital and give them more flexibility to develop new products, manufacturing processes and/or investments in other lines of business. Fewer parts in stock means fewer containers on the shop floor and more storage space.
Reducing bills of materials through 3D printing can reduce the cost of documentation, quality control, mass production planning and inventory management. Fewer parts means less time and labor to create the product itself, which leads to additional savings in production costs. nine0003
4. Creativity
The impressive potential of additive manufacturing cannot be realized without a large selection of materials. At the Jabil Materials Innovation Center in Chaska, Minnesota, designers, chemists, materials researchers and manufacturing experts interact with customers to develop high performance powders and filaments. These custom-designed materials enhance the performance, durability, fire resistance, conductivity and lubrication of the end product. nine0003
As Jabil's report shows, materials for 3D printing are on the rise, with plastics, polymers, composites and, with a slight lag, metals remaining the most common. Metal is less popular in 3D printing than plastic or polymer. This is the situation today, however, it may change, as survey participants said they would like to use more different materials in the future.
“Innovative materials don't just help increase bottom line. Revolutionary manufacturing approaches using new polymers can address important sustainability, healthcare, transportation, environmental and aerospace issues,” says Elizabeth Gardner, Jabil senior chemist and materials engineer. nine0003
The evolution of additive manufacturing
3D printing will develop dynamically, and in recent years we have seen how the pace of its implementation is growing. The convergence of digital technologies (including 3D scanning, integrated information systems and additive manufacturing) suggests the obvious: manufacturers are looking at digital solutions to increase profitability and accelerate the development and manufacture of their products.
This development will have implications for today's big business. “There is a lot of activity going on in this area, a lot of money and creative resources,” writes Richard D'Aveney in the Harvard Business Review, asking the question, “What is the price of waiting?” Indeed, new opportunities are increasing the speed and efficiency of 3D printing, and there is no doubt that successful companies would prefer to pick up this pace rather than slowly adopting the technology. nine0003
More and more companies are realizing the value and cost-effectiveness of additive technologies for design, prototyping and manufacturing. 3D printing is becoming one of the key tools in the arsenal of a manufacturing enterprise. If you use a 3D printer to streamline the iteration process and improve the product during the prototyping phase, you will ultimately save time and money, as well as reduce the error rate during the manufacturing phase. 3D printing is not just an innovation, it brings tangible benefits. nine0003
Research your customers first. It is essential to understand what they want and how best to meet their needs. 3D printing solutions are revolutionizing prototyping, tooling and product manufacturing. Large companies that actively use these opportunities (including design for additive manufacturing) will be in a better position and will be able to maintain stability.
D'Aveni recommends reviewing current processes. “3D printing is creating countless new approaches to manufacturing parts, and companies need to understand what supply chain configuration and what combination of old and new processes will become optimal,” the scientist argues. nine0003
Finally, the strategic implications need to be explored as AM will soon be adopted by entire trading platforms. Manufacturing is a 150-year-old industry that is inherently less subject to change. Nevertheless, we see that new technologies are being introduced more and more actively, and more than half of the top managers of companies that took part in the above survey see strategic opportunities in the use of additive technologies. In previous years, this percentage was lower. nine0003
The unique technologies, materials, processes and capabilities of additive manufacturing make it the cornerstone of digital transformation, a process that is already affecting many industries. There is no doubt that additive manufacturing is changing the way companies design and manufacture their products, and Jabil is an example of a business operating at the intersection of additive and conventional technologies.
Source: jabil.com
nine0002 Article published on 03.

3D prototyping of models, parts - additive technologies from DDM.Lab
Modern technologies allow you to create a really worthwhile product without spending a lot of development resources. 3D prototyping is the ability to produce an object according to a drawing or mathematical model in full size or scale, without losing the main functions of the final product. When using metals, thermoplastics, polymer blends and composites, high quality and functionality can be achieved without resorting to classical production. nine0003
Prototyping 3D models eliminates the risk of inaccurate design and engineering calculations and, consequently, unnecessary costs.
3D printing a model from a simplified (non-final) material or mass production material is the optimal solution for product development. You will be able to test a sample, check its functional properties and assembly compliance, make a presentation, and correct shortcomings even before the start of mass production. nine0003
Work stepsPrototyping typesTechnologiesPrices for 3D prototyping
Work steps
3D prototyping allows you to quickly start production in several basic steps: Testing the finished product, adjusting the digital model if necessary
With a prototype, you can evaluate its appearance, ergonomics, and even experience its functionality. If the object meets the requirements of the company, it can be used as a master model or tooling for the first batch of goods - in DDM.Lab you can order the production of products by molding into silicone (from 10 to 1000 pieces) or single- or reusable production tooling.
Prototypes are most often ordered by large machine-building and instrument-making companies, developers of innovative products and designers interested in the speedy release of plastic and metal products. Leave a request, and our experts will select for you the best way to introduce additive technologies into production! nine0003
Types of prototypes
Conventionally, prototypes can be divided into several types:
- Industrial - created for large manufacturing enterprises (assemblies, housings, parts and spare parts)
- Transport - such prototypes are used in mechanical engineering, aviation, shipbuilding (from development to individual parts or assemblies of land, water and air transport)
- Product prototypes allow you to work out the format of the future product and its packaging
- Presentation models, for example, a model of an apartment, a building or an entire city, make it possible to convey the project with its architectural features and interior design as clearly as possible.
for solving the problems of orthopedics, endoprosthesis, orthodontics.
Technologies
- FDM thermoplastic deposition modeling allows you to quickly and inexpensively produce fully functional samples of products and production tooling from a wide range of materials
- Inkjet printing with PolyJet photopolymers allows printing highly realistic color and multi-material products and tooling
- Selective laser fusion of LBM/SLM metals is often used for high-precision manufacturing of end products with complex geometry from metal-powder compositions of small fractions
- Gas-powder jet deposition using directed energy exposure DED/LMD is used for quick repair of worn surfaces of products, as well as for the manufacture of products from "scratch" from metal powder compositions
- 3DMP Arc Welding Wire Deposition - the most affordable and productive solution for the rapid production of high-stress end metal parts
- BJ inkjet printing by applying material powder and bonding it with a binder to create molds from sand-polymer compositions
- Selective SLS laser sintering for the rapid production of full-featured parts, production tooling from a wide range of polyamide and composite (polyamide-based) powders, and polystyrene powders for creating burnable master patterns for foundries
- SEAM screw extrusion hybrid additive manufacturing for rapid production of large-sized products using granular thermoplastics, including those filled with carbon fibers binder.
Learn more