3D printer tissue engineering
3D Bioprinting of Living Tissues
Progress in drug testing and regenerative medicine could greatly benefit from laboratory-engineered human tissues built of a variety of cell types with precise 3D architecture. But production of greater than millimeter sized human tissues has been limited by a lack of methods for building tissues with embedded life-sustaining vascular networks.
Play
In this video, the Wyss Institute and Harvard SEAS team uses a customizable 3D bioprinting method to build a thick vascularized tissue structure comprising human stem cells, collective matrix, and blood vessel endothelial cells. Their work sets the stage for advancement of tissue replacement and tissue engineering techniques.Multidisciplinary research at the Wyss Institute has led to the development of a multi-material 3D bioprinting method that generates vascularized tissues composed of living human cells that are nearly ten-fold thicker than previously engineered tissues and that can sustain their architecture and function for upwards of six weeks. The method uses a customizable, printed silicone mold to house and plumb the printed tissue on a chip. Inside this mold, a grid of larger vascular channels containing living endothelial cells in silicone ink is printed, into which a self-supporting ink containing living mesenchymal stem cells (MSCs) is layered in a separate print job. After printing, a liquid composed of fibroblasts and extracellular matrix is used to fill open regions within the construct, adding a connective tissue component that cross-links and further stabilizes the entire structure.
Confocal microscopy image showing a cross-section of a 3D-printed, 1-centimeter-thick vascularized tissue construct showing stem cell differentiation towards development of bone cells, following one month of active perfusion of fluids, nutrients, and cell growth factors.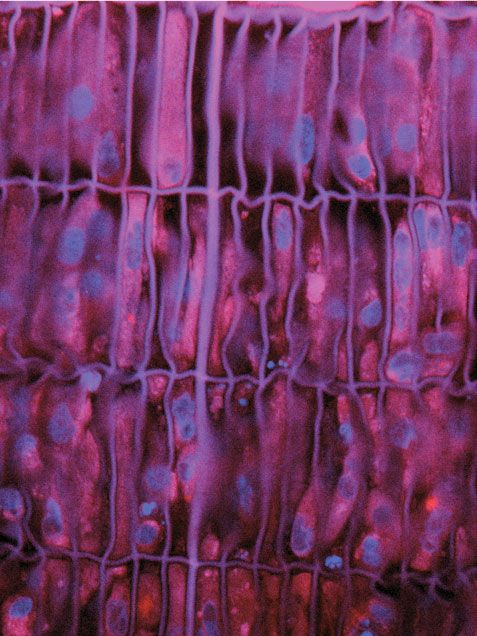
The resulting soft tissue structure can be immediately perfused with nutrients as well as growth and differentiation factors via a single inlet and outlet on opposite ends of the chip that connect to the vascular channel to ensure survival and maturation of the cells. In a proof-of-principle study, one centimeter thick bioprinted tissue constructs containing human bone marrow MSCs surrounded by connective tissue and supported by an artificial endothelium-lined vasculature, allowed the circulation of bone growth factors and, subsequently, the induction of bone development.
This innovative bioprinting approach can be modified to create various vascularized 3D tissues for regenerative medicine and drug testing endeavors. The Wyss team is also investigating the use of 3D bioprinting to fabricate new versions of the Institute’s organs on chips devices, which makes their manufacturing process more automated and enables development of increasingly complex microphysiological devices. This effort has resulted in the first entirely 3D-printed organ on a chip – a heart on a chip – with integrated soft strain sensors.
- 1/7 Cross section of long-term perfusion of HUVEC-lined (red) vascular network supporting HNDFladen (green) matrix.
- 2/7 Top-down view of long-term perfusion of HUVEC-lined (red) vascular network supporting HNDFladen (green) matrix.
- 3/7 Photograph cross section of printed tissue construct housed within a perfusion chamber.
- 4/7 Photograph cross section of printed tissue construct housed within a perfusion chamber.
- 5/7 Photograph of a printed tissue construct housed within a perfusion chamber.
- 6/7 Photograph of vasculature network and cell inks.
- 7/7 Photograph of 3D printed vasculature network (red) within Red is the
- Next
- Prev
3D printing in tissue engineering: a state of the art review of technologies and biomaterials
To read this content please select one of the options below:
Nataraj Poomathi (Department of Mechanical Engineering, Centre for Nanofibers and Nanotechnology Initiative, National University of Singapore, Singapore and Organic and Bioorganic Chemistry Division, CSIR-Central Leather Research Institute, Chennai, India)
Sunpreet Singh (Department of Mechanical Engineering, National University of Singapore, Singapore)
Chander Prakash (School of Mechanical Engineering, Manufacturing Domain, Lovely Professional University, Phagwara, India)
Arjun Subramanian (Centre for Nanofibers and Nanotechnology Initiative, Department of Mechanical Engineering, National University of Singapore, Singapore)
Rahul Sahay (Division of Engineering Product Development, Singapore University of Technology and Design, Singapore)
Amutha Cinappan (Department of Mechanical Engineering, National University of Singapore, Singapore)
Seeram Ramakrishna (Department of Mechanical Engineering, National University of Singapore, Singapore)
Rapid Prototyping Journal
ISSN: 1355-2546
Article publication date: 24 June 2020
Issue publication date: 23 July 2020
Downloads
Abstract
Purpose
In the past decade, three-dimensional (3D) printing has gained attention in areas such as medicine, engineering, manufacturing art and most recently in education. In biomedical, the development of a wide range of biomaterials has catalysed the considerable role of 3D printing (3DP), where it functions as synthetic frameworks in the form of scaffolds, constructs or matrices. The purpose of this paper is to present the state-of-the-art literature coverage of 3DP applications in tissue engineering (such as customized scaffoldings and organs, and regenerative medicine).
Design/methodology/approach
This review focusses on various 3DP techniques and biomaterials for tissue engineering (TE) applications. The literature reviewed in the manuscript has been collected from various journal search engines including Google Scholar, Research Gate, Academia, PubMed, Scopus, EMBASE, Cochrane Library and Web of Science. The keywords that have been selected for the searches were 3 D printing, tissue engineering, scaffoldings, organs, regenerative medicine, biomaterials, standards, applications and future directions. Further, the sub-classifications of the keyword, wherever possible, have been used as sectioned/sub-sectioned in the manuscript.
Findings
3DP techniques have many applications in biomedical and TE (B-TE), as covered in the literature. Customized structures for B-TE applications are easy and cost-effective to manufacture through 3DP, whereas on many occasions, conventional technologies generally become incompatible. For this, this new class of manufacturing must be explored to further capabilities for many potential applications.
Originality/value
This review paper presents a comprehensive study of the various types of 3DP technologies in the light of their possible B-TE application as well as provides a future roadmap.
Keywords
- 3D printing
- Biomaterial
- Biomedical engineering
- Regenerative medicine
- Organs
- Tissue
- Scaffoldings
- Standards
Acknowledgements
One of the authors Dr N. Poomathi thanks the Department of Science and Technology (DST), New Delhi for the award of the Women Scientist Fellowship under women scientist scheme (WOS-A).
Citation
Poomathi, N., Singh, S., Prakash, C., Subramanian, A., Sahay, R., Cinappan, A. and Ramakrishna, S. (2020), "3D printing in tissue engineering: a state of the art review of technologies and biomaterials", Rapid Prototyping Journal, Vol. 26 No. 7, pp. 1313-1334. https://doi.org/10.1108/RPJ-08-2018-0217
Publisher
:Emerald Publishing Limited
Copyright © 2020, Emerald Publishing Limited
Related articles
3D printing of human organs: how it works
“Will we finally get new organs printed?” - this strange question in our days, it turns out, is already in the air. So, we inform you: they will print it. But not now. Not so soon. Although bioprinters are already being developed in Russia, which in the future will be used to print “spare parts” for humans, and biopaper for such devices.
One of such domestic "points of growth" is the Laboratory of Tissue Engineering of the Institute of Theoretical and Experimental Biophysics (ITEB RAS), located in the science city of Pushchino near Moscow. nine0003
What does the term “tissue engineering” mean and where did it come from?
Before making new kidneys and a heart from scratch (which we still do not know how), medicine had to master two simpler tasks. First, learn to reproduce hard tissues - bones. And secondly, to learn how to recreate large pieces of tissue to "patch" severe injuries.
This is going pretty well so far. In both cases, "biodegradable materials" are used. They do not remain in the body forever, but form the basis, populating which, human stem cells gradually restore the tissue. In this case, the “patch” itself simply dissolves. nine0003
First of all, MIR 24 correspondents were shown something that looked like "sugar cubes" in flasks. As it turned out, these are stocks of materials or preparations from which a bone substitute is formed in humans. "White matter" can be either natural bone or synthetic polymers such as polylactides and polyglycolides.
Computer-controlled 3D-printed bone tissue in its structure can both completely recreate the lost bone fragment and create other structures suitable for ensuring the process of its restoration. nine0003
“The possibilities of 3D bioprinting have made it possible, for example, to replace a human lower jaw removed due to a cancerous tumor,” says Irina Selezneva, head of the Cell and Tissue Growth Laboratory. “Before removing it, they took a tomogram and, using a computer model, restored and printed the frame of the organ, which was then populated with the patient’s own stem cells and replaced the loss.”
With the reproduction of soft tissues, the situation is more complicated. However, over the past ten years, scientists have made significant progress in this direction. nine0003
The essence of the "bioprinting" method in this case is that the future organ is formed from two main components: living cells and a "matrix" that simulates the conditions of the intercellular environment and connective tissue.
The source of cells can be both donor and own human stem cells isolated, for example, from fat or bone marrow. They can be transformed into various types of cells and tissues under the influence of biologically active substances.
Head of the Laboratory of Tissue Engineering Professor Vladimir Akatov and Irina Selezneva talk about the creation of new bioactive materials capable of activating the body's own regenerative capabilities without cells introduced from outside. The main thing is to create conditions for the migration and growth of human stem cells and the formation of tissues by them. nine0003
“Biopaper for a bioprinter” scientists call an artificial environment in which living cells of future organs can grow. It is formed from proteins, polysaccharides and other bioactive substances and is a hydrogel that can be filled into a bioprinter along with cells, or a thin film on which cells can be printed.
“We are testing these gels by interacting with cells,” explains Senior Researcher Galina Davydova. “We are looking at how to compose a composition so that, after polymerization, the hydrogel provides the mechanical characteristics of the structure and the conditions for life in them. ” nine0003
Galina Anatolyevna draws collagen protein into one syringe, and polysaccharide (methylcellulose) into the other. And drips from both syringes into a Petri dish. A reaction occurs, resulting in a shapeless "foam" or film in the cup. It resembles paper very relatively - however, something like a piece of embossed wallpaper or linkrust. This gel "polymerizes".
Here is the prototype of the "substrate" where the cells of future organs will grow layer by layer. It will be able to form three-dimensional three-dimensional structures of these organs, and then, having played its role, will be absorbed in the body. So far, nothing stunning in appearance does not resemble. nine0003
However, Pushchi residents have quite solid partners. “In our country, there are two leaders in bioprinting, somewhat different in their approaches and hardware,” said Irina Ivanovna Selezneva. “One of them is Vladimir Mironov, head of 3D Bioprinting Solutions and a professor at the University of Virginia. ”
Mironov's technology is similar to an "inkjet printer", when, under computer control, jets from different syringes are mixed, forming a fabric on the substrate. “Cell spheroids are used as ink, cell aggregates that have the ability to merge with each other, forming the same capillaries and other structures, tissues,” Selezneva noted. nine0003
The other leader is Boris Chichkov, professor at Hannover University. Leibniz and head of the laboratory of laser nanoengineering at the Institute for Problems of Laser and Information Technologies of the Russian Academy of Sciences in Troitsk.
“Let's call it laser bioprinting,” Selezneva said. - Very short, femtosecond laser pulses make it possible to stitch the material step by step, setting the desired matrix shape under computer control with an accuracy of nanometers. The same laser pulses are capable of transferring even individual cells from one surface to another, which at the same time retain their viability. ” nine0003
Bioprinting technologies differ, but without a matrix that provides an adequate microenvironment for cell life and tissue formation, in both cases it is indispensable. Pushchino is developing “paper” for both inkjet and laser printers, adapting the characteristics of hydrogels to the characteristics of bioprinting technology.
In principle, using bioprinting methods in the distant future, it may be possible to assemble an organ like a puzzle from individual cells and a matrix. And in the near future, tissue pieces printed in this way will become a new model for testing new drugs. nine0003
The most important task that scientists set for the future is to learn how to build up tissue right on the damaged area. Then, instead of a bulky printer, a tool like a gun will be used, from which hydrogel elements with cells will be applied to the patient's body, which will polymerize directly on the person, forming a new tissue. Leonid Smirnov
0003 Study demonstrated viability fabric frames printed on a 3D printer, which are harmless are destroyed, contributing to tissue regeneration after implantation, - writes new. Scaffolds have shown promising tissue healing properties, including the ability to support cell migration, "ingrowth" tissue and revascularization (growth of blood vessels). nine0003 Professor Andrew Dove of the School of Chemistry at the University of Birmingham led the research team and is the lead author article published in Nature Communications, in which describe the physical properties of the frameworks and explain how they "shape memory" is the key to promoting tissue regeneration. Professor Dove commented: “The frameworks have uniform distributed and interconnected pores that provide diffusion of nutrients from surrounding tissues. shape memory means that this structure is preserved when the framework implanted in tissues and this supports cell infiltration into scaffold while encouraging tissue regeneration and revascularization." nine0003 The frameworks were created using 3D printing resins, developed during a major research program biomaterials, conducted by Professor Andrew Dove from the University Birmingham and the University of Warwick. Frameworks have shown several major advantages over existing approaches used to fill voids in soft tissues that remain after injury or surgery interference, including sufficient elasticity to conform to uneven spaces, the ability to be exposed compression up to 85% before returning to its original geometry, fabric compatibility and non-toxic biodegradation. nine0003 This article describes several formulations of 4Degra™ resins that allow the production of materials with a wide range of strengths. All compositions include a photoinitiator and a photoinhibitor to resins quickly gelled when exposed to light in the visible spectrum, which allows them to be 3D printed on frames of various geometry. Researchers have shown that the materials are not toxic to cells, and they also performed mechanical tests to ensure that scaffolds can restore their shape, geometry and pore size after compression, and also conducted tests that showed that scaffolds can fill irregularly shaped voids in alginate gel, which was used as an imitation of soft tissues. Laboratory studies have shown that the scaffold decomposes into the result of surface erosion on non-acidic products, which means that the frame structure provides slow and continuous tissue infiltration. The results were confirmed in a mouse model that mimics implantation in adipose tissue. These studies have shown infiltration of adipocytes and fibroblasts and vascularization after two months, as well as the location of tissues and the presence of macrophages, which indicated the restoration of normal tissue, and not about damaged, scarred tissue or an inflammatory reaction. nine0003 Four months later, the researchers found in the surrounding tissue small mature blood vessels. Frames also demonstrated excellent biocompatibility. Collagen the capsule formed around the implants had a thickness less than 200 µm, well below the 500 µm threshold used for determination of biocompatibility in other studies, and was not calcification or necrosis. Also after four months, 80% of the scaffold was still present, demonstrating the slow degradation predicted by laboratory research, and indicates that the scaffolds will provide support for more than a year, providing enough time for germination of mature tissue. The controls that used poly (L-lactic acid) (PLLA) as a reference, not showed a significant decline over a four-month period. nine0003 Professor Dove comments: “3D printed materials are much attention in the world of tissue engineering. However, the materials fill voids, provide mechanical support, biocompatibility and surface erosion characteristics that provide continuous tissue support during the healing process, and this means that the fourth dimension (time) is needed take into account in material design. We have demonstrated that it is possible to produce highly porous frameworks with shape memory, and our processes and materials will enable the production of self-selecting scaffolds that accept the geometry of soft tissue voids when minimally invasive surgery without deformity or pressure on surrounding tissues. 3D printed scaffolds support tissue growth after implantation
eurekalert.org.
Resins are sold under trade brand 4Degra™ by 4D Biomaterials, a subsidiary of University of Birmingham Enterprise and Warwick Innovations, which was launched in May 2020.
nine0003